They’re powerful, they’re fast, and we aren’t sure about what causes them, but astronomers are closer than ever to understanding the source of mysterious fast radio bursts.
Flashes Without a Cause
Fast radio bursts: one of the most recent mysteries to appear in the sky and one of the most active fields of astronomical research. Since the discovery of the first of these powerful <1-second eruptions eruptions of radio waves back in 2007, astronomers have recorded hundreds of similar events. We know that they must originate from beyond our Milky Way galaxy, but, beyond that, astronomers have still yet to settle on a consensus about what might cause such brief, energetic flashes. This mystery of the origins of these bursts has driven many astronomers into an exciting, frustrating, and increasingly productive quest to understand whatever immense forces power them.
It is not easy to study a flash; by their very nature, they appear for only a fraction of a second, then vanish to almost never return. While a precious few do eventually repeat, they do so at largely irregular intervals, meaning astronomers can never really be sure when or where a fast radio burst might happen. The ones astronomers do manage to spot are almost always flagged by survey telescopes that scan huge swaths of the sky at once. A wide field of view comes with a tradeoff, though: although these telescopes can monitor enough sky that they have a good chance of catching a burst, their view of the sky is fairly blurry. So, although astronomers have recorded a few hundred bursts by now, they usually can’t say exactly where each one came from.
For the 16 years since the discovery of the first fast radio burst, astronomers have been trying to piece together their secrets without even knowing the location of each flash. Recently, however, they have made progress both in narrowing in on their quarry and on understanding their source. Below are three recent studies published in AAS Journals detailing this progress.
Zooming In
First up is a study published in May of this year by a team led by Shivani Bhandari, Netherlands Institute for Radio Astronomy. Bhandari and collaborators describe their discovery of a fast radio burst using the Australian Square Kilometre Array Pathfinder, a relatively new and phenomenally capable radio telescope that they used the pin down the location of the flash to within one arcsecond (about 0.03% of a degree!). This extreme precision allowed the team to identify which galaxy the flash came from, and what they found was somewhat surprising: the host was a small, somewhat boring dwarf galaxy with almost no ongoing star formation. This is in contrast to the handful of known hosts of repeating fast radio burst, which were all more lively, active galaxies. Considering both the host and the properties of the burst itself, the team concluded that their burst could have been caused by an “accretion jet from a hyperaccreting black hole.”
Magnetar Earthquakes?
A month later in early June, a team led by Fayin Wang, Nanjing University, published their own analysis of archival data to suggest an alternative source. By digging through all of the observations of two known repeating fast radio burst collected by the Five-hundred-meter Aperture Spherical radio Telescope (FAST), Wang and colleagues realized that the gaps between bursts were not quite as random as previously thought. Instead, whatever was causing the bursts seemed to have “memory,” meaning the triggers must be correlated in time. Building from this, they advocate for a different explanation, positing that the bursts occur whenever a highly magnetized neutron star undergoes “crustal fractures” — in other words, earthquakes. After a shift, the magnetic stresses will build up again and cause the process to repeat, which could give rise to the recurring bursts.
More Than One
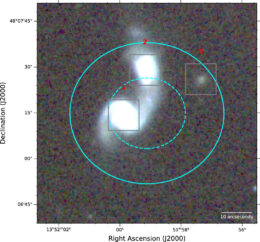
The location of one of the thirteen repeating fast radio burst, which lines up perfectly with a pair of merging spiral galaxies. [Michilli et al. 2023]
Finally, in late June, another study led by Daniele Michilli, Massachusetts Institute of Technology, offered a bridge between the two previous ones. This publication describes a re-analysis of data collected by the Canadian Hydrogen Intensity Mapping Experiment (CHIME), which resulted in newer, much more precise estimates of previous fast radio burst locations. The team focused on 13 repeating bursts and pinned down each of their locations to within about 10 arcseconds. While that isn’t precise enough to nail the host galaxy for all 13, they did mange to conclusively identify the host for two of them. Intriguingly, these two galaxies were nothing alike: one is a peaceful, quiescent galaxy, and the other is one in a pair of merging spiral galaxies that are actively forming many stars. This suggests that fast radio burst can come from a range of environments, or even that there could be multiple causes that each produce a similar looking signal.
While the final, well-supported model to describe all fast radio burst is still out of reach, astronomers are actively getting closer to this final goal. As new telescopes and processing techniques come online, it is only a matter of time until enough data is collected and analyzed that a clearer picture emerges. Soon, what now appear as mysterious flashes will be the subjects of well-documented chapters in the next textbooks, and this knowledge will be based on studies happening today, like these three.
Citation
“A Nonrepeating Fast Radio Burst in a Dwarf Host Galaxy,” Shivani Bhandari et al 2023 ApJ 948 67. doi:10.3847/1538-4357/acc178
“Repeating Fast Radio Bursts Reveal Memory from Minutes to an Hour,” F. Y. Wang et al 2023 ApJL 949 L33. doi:10.3847/2041-8213/acd5d2
“Subarcminute Localization of 13 Repeating Fast Radio Bursts Detected by CHIME/FRB,” Daniele Michilli et al 2023 ApJ 950 134. doi:10.3847/1538-4357/accf89