In astronomy, not detecting something can tell us something useful. A recent article details a radio search for six supernovae that resulted in no detections — but still gives us hints about the companions of these exploding stars.
How Stars Explode
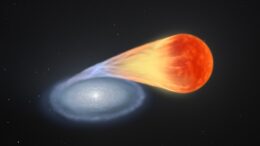
Accretion from a companion star onto a white dwarf is one way to trigger a supernova. [Still image from an animation by NASA’s Goddard Space Flight Center Conceptual Image Lab]
Researchers suspect that nearly any type of star, from compact white dwarfs to puffed-up supergiants, can donate mass to a white dwarf and trigger a supernova. Which stars actually do is an active area of research, especially since these explosions are useful cosmic distance markers. But how can we tell from the aftermath of the explosion what kind of star was involved?
A Rapid Radio Search
When a star donates mass to its white dwarf companion, some of that gas remains in the space between the two stars, and the distribution of the gas can tell us about the type of companion star; for example, the billowing stellar winds of a supergiant should create an extended region of low-density gas. When a supernova’s shock wave collides with and compresses this gas, it generates synchrotron radiation from electrons traveling in helical paths around magnetic field lines. Synchrotron radiation is produced even when the supernova collides with very low-density material that would be impossible to see through other means.
Chelsea Harris (Michigan State University) and collaborators performed a search for synchrotron radiation from six nearby supernovae that had been detected at optical wavelengths. Judging by the evolution of their optical light curves, these supernovae all resulted from exploding white dwarfs with mass-donating stellar companions. However, the team found no radio emission to accompany the rapidly fading optical light of any of the supernovae in their sample.
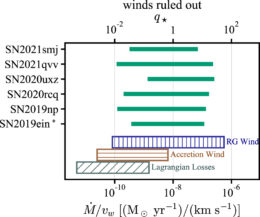
Non-detections of the six supernovae rule out winds with the parameters spanned by the green bars. Click to enlarge. [Harris et al. 2023]
Winds, Shocks, and Shells
By modeling how much radio emission we’d expect to see from various distributions of circumstellar gas, the team was able to rule out the presence of low-density stellar winds from a supergiant companion. The non-detections also ruled out most winds due to accretion of material onto the white dwarf.
As part of their investigation, Harris and collaborators also modeled the expected synchrotron emission from a shock wave colliding with a dense shell of circumstellar gas. This scenario might arise when a pre-supernova white dwarf undergoes one or more novae before the ultimate explosion. Unexpectedly, the team found that these dense shells probably don’t produce a detectable amount of synchrotron emission. While radio observations are a powerful tool to study circumstellar gas, these shells might make themselves known on the other side of the electromagnetic spectrum, with fleeting bursts of X-rays or gamma rays.
Citation
“Radio Observations of Six Young Type Ia Supernovae,” C. E. Harris et al 2023 ApJ 952 24. doi:10.3847/1538-4357/acd84f