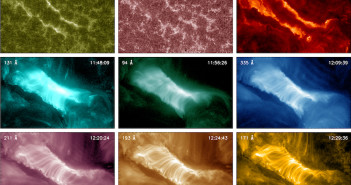
Selections from 2016: A Connection Between Solar Explosions and Dimming on the Sun
Editor’s note: In these last two weeks of 2016, we’ll be looking at a few selections that we haven’t yet discussed on AAS Nova from among the most-downloaded papers published in AAS journals this year. The usual posting schedule will resume after the AAS winter meeting.
The Nature of CME-Flare-Associated Coronal Dimming
Published June 2016
Main takeaway:
The Solar Dynamics Observatory (SDO) observed a large solar eruption at the end of December 2011. Scientists Jianxia Cheng (Shanghai Astronomical Observatory and the Chinese Academy of Sciences) and Jiong Qiu (Montana State University) studied this coronal mass ejection and the associated flaring on the Sun’s surface. They found that this activity was accompanied by dimming in the Sun’s corona near the ends of the flare ribbons.
Why it’s interesting:
The process of coronal dimming isn’t fully understood, but Cheng and Qiu’s observations provide a clear link between coronal dimming and eruptions of plasma and energy from the Sun. The locations of the dimming — the footpoints of the two flare ribbons — and the timing relative to the eruption suggests that coronal dimming is caused by the ejection of hot plasma from the Sun’s surface.
How this process was studied:
There are a number of satellites dedicated to observing the Sun, and several of them were used to study this explosion. Data from SDO’s Atmospheric Imaging Assembly (which images in extreme ultraviolet) and its Helioseismic and Magnetic Imager (which measures magnetic fields) were used — as well as observations from STEREO, the pair of satellites orbiting the Sun at ±90° from SDO.
Citation
J. X. Cheng and J. Qiu 2016 ApJ 825 37. doi:10.3847/0004-637X/825/1/37