High in the Sun’s atmosphere, solar storms are brewing. As magnetic fields twist and coil, they build up enough energy to unleash flares and massive outbursts of plasma into the solar system. For the sake of the thousands of satellites in Earth’s orbit and the well-being our astronauts, we’d like to know when that’s going to happen. How can we predict space weather events?
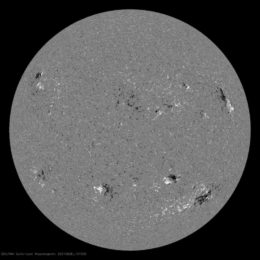
An example of a magnetic field map from Solar Dynamics Observatory. The black areas indicate magnetic field lines that point away from Earth, while the white areas indicate magnetic field areas pointing toward Earth. Each pair of black and white areas roughly corresponds to the location of a sunspot. [NASA/SDO/GSFC]
Signs on the Sun’s Surface
To predict inclement space weather before it happens, scientists need to know the strength and configuration of the magnetic field in the Sun’s upper atmosphere — its chromosphere and corona — where magnetic energy builds up and is released in the form of solar flares and immense eruptions of solar plasma called coronal mass ejections. This region is difficult to observe, so most space weather forecasts use measurements of the magnetic field lower in the Sun’s atmosphere, in the photosphere, instead.
Although these photospheric measurements are plentiful, they’re too far removed from the region where space weather is launched to yield accurate forecasts. In today’s article, a team led by Philip Judge (High Altitude Observatory, National Center for Atmospheric Research) explores other ways to probe the magnetic field structure of the Sun’s upper atmosphere and make better space weather predictions.
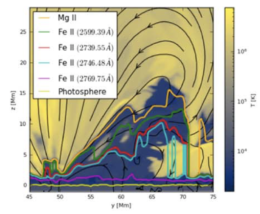
Simulation of an emerging system of magnetic flux (blue region). The colored lines indicate the altitude at which the individual spectral lines of magnesium and iron form. The simulated spectral lines all probe regions well above the solar photosphere, with the magnesium line probing the highest altitudes. Click to enlarge. [Adapted from Judge et al. 2021]
Precisely Measured Photons
Judge and collaborators combined archival data with simulations to analyze the solar spectrum, hunting for wavelengths emitted by the hot plasma in the Sun’s chromosphere and corona. The authors were interested in finding emission lines sensitive to the Zeeman effect, in which the presence of a magnetic field splits spectral lines into multiple components, and the Hanle effect, in which the presence of a magnetic field randomizes the orientations of the electric fields of the emitted light (in other words, this effect reduces the polarization of the emissions).
The authors highlighted near-ultraviolet emissions from magnesium and iron in the 256–281-nm range as the best for studying the sources of solar eruptions, since these wavelengths arise from high in the Sun’s atmosphere. By modeling the polarization of magnesium lines in this wavelength range, Judge and collaborators demonstrate that observations of this portion of the Sun’s spectrum can probe a large range of magnetic field strengths and orientations — key for predicting when and where solar flares and coronal mass ejections will occur.
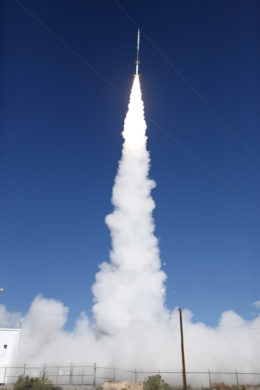
CLASP-2 made the first measurement of polarized emission from magnesium at ultraviolet wavelengths. [US Army]
A New Eye on the Sun?
Although the authors demonstrate that this wavelength range is a promising window into the Sun’s magnetic field configuration, exploring it requires a space telescope to perform an exacting technique called spectropolarimetry — measuring the polarization of individual wavelengths of light — and extract the desired magnetic-field information.
Though this technique is relatively new in solar physics, Judge and coauthors point to a 2019 sounding-rocket experiment, Chromospheric LAyer SpectroPolarimeter 2 (CLASP-2), which made spectropolarimetric measurements of the Sun during the 6 minutes it was high in Earth’s atmosphere, as a proof of concept. The authors suggest that even a small (~25-cm) space telescope would suffice, greatly improving our ability to forecast space weather events.
Citation
“Measuring the Magnetic Origins of Solar Flares, Coronal Mass Ejections, and Space Weather,” Philip Judge et al 2021 ApJ 917 27. doi:10.3847/1538-4357/ac081f