Editor’s Note: Astrobites is a graduate-student-run organization that digests astrophysical literature for undergraduate students. As part of the partnership between the AAS and astrobites, we occasionally repost astrobites content here at AAS Nova. We hope you enjoy this post from astrobites; the original can be viewed at astrobites.org.
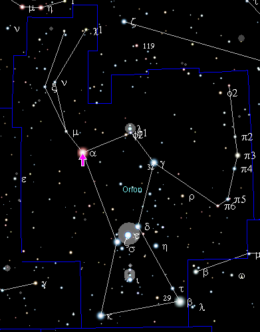
Figure 1: A schematic of the Orion constellation, with a small pink arrow indicating the location of Betelgeuse in Orion’s left shoulder. Click to enlarge. [Wikipedia]
Red supergiants like Betelgeuse are known to experience radial pulsations: repeated expansion and contraction of the star’s outer layers that cause periodic fluctuations in brightness. Like standing waves on a string, these pulsations include both fundamental and higher-order modes, with the fundamental mode dictating the longest possible pulsation period. For Betelgeuse, astronomers have measured a fundamental-mode period of about 420 days. However, they have also observed a long secondary period of 2,100 days, the origin of which is a mystery. Or is it?
Today’s bite covers two articles that independently reach the same conclusion: Betelgeuse’s long secondary period is caused by the presence of a low-mass binary companion! Such a small star would be drowned out by Betelgeuse’s intrinsic brightness and variability, explaining why we haven’t detected it before. But as today’s articles show, careful analysis of radial velocity and astrometry data can tease out signatures of Betelgeuse’s hidden buddy.
Article 1: One Long-Secondary-Period Mechanism to Rule Them All
Title: A Buddy for Betelgeuse: Binarity as the Origin of the Long Secondary Period in α Orionis
Authors: Jared A. Goldberg, Meridith Joyce, and László Molnár
First Author’s Institution: Flatiron Institute
Status: Published in ApJ
In Article 1, the authors use light curves from the American Association of Variable Star Observers (AAVSO) and radial velocities from STELLA to test the plausibility of eight different explanations for Betelgeuse’s long secondary period. Light curves are a record of how a star’s brightness (or magnitude) changes over time, and radial velocities record how quickly a star is moving towards or away from us. For Betelgeuse, both datasets show the ~2,100-day long secondary period. The authors measure a phase offset between the datasets of about −2 radians, meaning that the radial velocity curve lags behind the light curve by about half an orbit. With these data in hand, they are able to rule out all proposed long-secondary-period mechanisms that don’t involve a binary companion. For example:
- Is the long secondary period really the fundamental mode? No! If this were the case, Betelgeuse would need to have a much larger radius than what we’ve measured. The ~420-day fundamental mode aligns much more closely with our observations.
- Is the long secondary period caused by the motion of convective cells on Betelgeuse’s surface? No! Convection would introduce random variability that we don’t observe. Plus, we expect convective motions on the surfaces of all luminous giant stars, but we know that not all of these stars show long secondary periods like Betelgeuse does.
- Is the long secondary period caused by starspots coming in and out of view? No! Betelgeuse’s long-secondary-period variations are stronger at bluer wavelengths than redder wavelengths, which starspots can’t replicate. We’d also expect Betelgeuse’s magnetic activity cycle to have a longer period than the long secondary period.
The authors conclude the long secondary period is most likely caused by binarity. However, a small companion passing in front of Betelgeuse would not cause noticeable dimming compared to the variability from pulsations. Since red supergiants like Betelgeuse have dusty circumstellar environments, previous work suggested that a hypothetical companion could drag a cloud of dust along with it to produce more significant dimming. This theory would also explain why long-secondary-period variations are stronger at bluer wavelengths, where dust scatters more light than at redder wavelengths.
There’s just one problem: the measured phase offset of −2 radians suggests that the companion would be in front of Betelgeuse when the system reaches maximum brightness, not behind it as the above theory suggests. To address this, the authors propose that rather than dragging dust along, the companion somehow destroys or modifies dust to cause an increase in Betelgeuse’s brightness as it passes in front of the larger star (see Figure 2). However, they note that future work is necessary to determine exactly how this could occur.
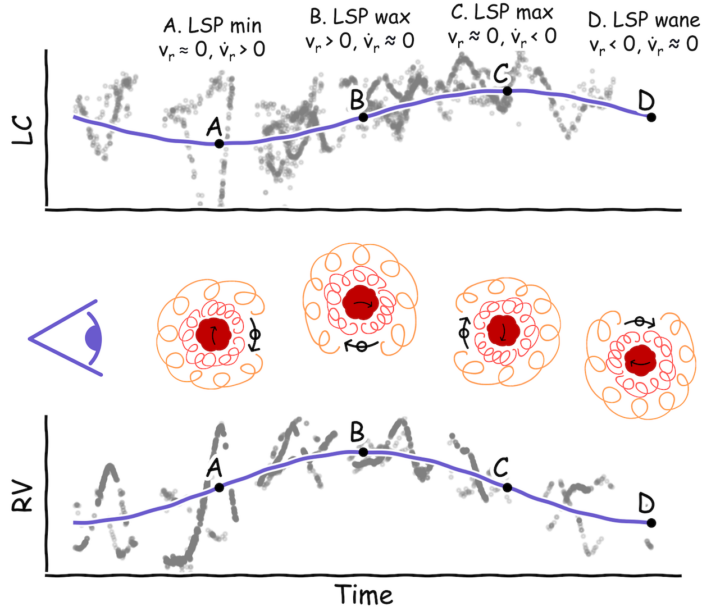
Figure 2: A sketch showing how Betelgeuse’s light curve variability (top graph) and radial velocity variability (bottom graph) can be explained by the presence of a binary companion. The light curve is noticeably out of phase with the radial velocity curve, as found in both articles. The middle row of drawings shows what an observer on Earth would see at each of the four points labeled on the two graphs. Betelgeuse is shown as a red circle, its hypothetical companion is shown as a black circle, and its circumstellar dust is shown as curly orange and red lines. Note that at maximum brightness (Point C), the companion is in front of Betelgeuse instead of behind it. [Goldberg et al. 2024]
Article 2: Finding Binarity in a Century’s Worth of Data
Title: Radial Velocity and Astrometric Evidence for a Close Companion to Betelgeuse
Authors: Morgan MacLeod et al.
First Author’s Institution: Center for Astrophysics | Harvard & Smithsonian; Institute for Theory and Computation
Status: Published in ApJ
In Article 2, the authors leverage more than 100 years of radial velocity data — including the radial velocities from Article 1 and several other, independent datasets — to search for a binary companion to Betelgeuse. First, they construct a model including the unseen companion, a flexible Gaussian process kernel to represent variability from other sources, and a noise term. Then, they fit their radial velocities with this model to retrieve the period, phase, and amplitude of the long-secondary-period signal (see Figure 3).
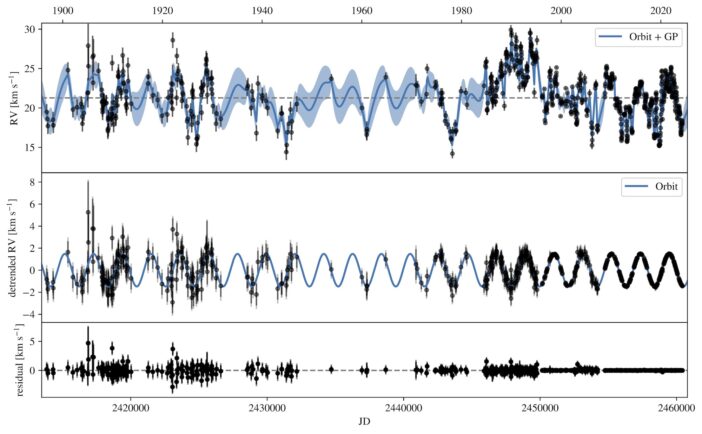
Figure 3: Results of fitting a model (blue lines) to 100 years of radial velocity data (black points). The top panel shows the full model, including the flexible kernel used to fit all variability sources other than the long secondary period (labeled “GP” here for “Gaussian process”). The middle panel shows just the sinusoidal component of the fit that represents the long secondary period, which remains a good fit over the whole baseline. The bottom panel shows the residuals after the full model (from the top panel) is subtracted from the data. [MacLeod et al., in press]
Using their fitted parameters, the authors derive a mass of 0.60 ± 0.17 M☉ and an orbital separation of 1,818 ± 6 R☉ for Betelgeuse’s hidden companion. This mass is significantly smaller than the estimate from Article 1, which the authors attribute to differences in the fitted long-secondary-period amplitudes between the articles. Betelgeuse’s radial velocity curve includes multiple sources of variability from radial pulsations, the signature from the binary companion, and other random changes. As a result, the true amplitude of the long-secondary-period variations is highly uncertain, even when the period is well constrained.
In addition to radial velocities, the authors also investigate Betelgeuse’s long secondary period with astrometry. Astrometric data record the relative positions and motions of the stars, which are expected to follow predictable patterns. An unseen binary companion will introduce an extra “wobble” in this pattern by tugging the visible star back and forth as it moves through space. These wobbles can be modeled to determine the orbital parameters of the binary, which should agree with the parameters derived from radial velocity data.
The authors fit a model to their astrometry and find two prominent periods: 1) the long secondary period at ~2,100 days and 2) an unexpected periodicity at ~1,650 days (about four times the fundamental mode). They also find a variability amplitude that is higher than their fitted radial velocity amplitude, implying a companion mass of 2.1 ± 0.5 M☉. However, their data are fit equally well by a binary model or a single-star model that includes a significant noise term. This is likely due to the fact that Betelgeuse’s radial extent is larger than its expected astrometric wobble, making precise astrometric measurements difficult. The authors conclude that additional observations are needed to constrain the binarity of Betelgeuse with astrometry, noting that their radial velocity results are more trustworthy given the longer baseline, higher cadence, and higher precision of the radial velocity data.
Using their measured parameters of the Betelgeuse system, the authors calculate the evolution of the hypothetical binary due to tides. Just like the Moon’s gravitational pull creates tides on Earth, the stars in a binary system pull on each other as well, dissipating angular momentum from the system and moving closer together in a process called “orbital decay.” In the case of Betelgeuse, the authors find that tides will cause runaway orbital decay over the next 10,000 years, where the system will be unable to stabilize as the orbital separation shrinks. This means Betelgeuse will interact with and eventually swallow its tiny companion!
Staying Hidden… for Now
Despite using different datasets and fitting methods, both of today’s articles find evidence that the ~2,100-day long secondary period in Betelgeuse’s light curve is likely due to the presence of a previously undetected, low-mass binary companion. Direct detection of such a companion is nearly impossible with current instruments, but further study of the dust around Betelgeuse could help constrain the companion’s properties. If confirmed, Betelgeuse’s binarity would have significant implications for both its evolution and for other evolved stars with long secondary periods, which may also host hidden binary buddies!
Original astrobite edited by Lindsey Gordon.
About the author, Alexandra Masegian:
Alexandra is a second-year PhD student in astronomy at Columbia University and the American Museum of Natural History. She is broadly interested in stellar astrophysics, especially evolved stars and binaries. Outside of work, she enjoys cooking, reading and writing science fiction, and visiting national parks.