Now that we’re able to detect gravitational waves, the next challenge is to spot electromagnetic signatures associated with gravitational-wave events. A team of scientists has proposed a new algorithm that might narrow the search.
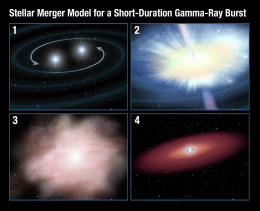
Artist’s illustrations of the stellar-merger model for short gamma-ray bursts. In the model, 1) two neutron stars inspiral, 2) they merge and produce a gamma-ray burst, 3) a small fraction of their mass is flung out and radiates as a kilonova, 4) a massive neutron star or black hole with a disk remains after the event. [NASA, ESA, and A. Feild (STScI)]
Light from Neutron-Star Mergers
Just over a year ago, LIGO detected its first gravitational-wave signal: GW150914, produced when two black holes merged. While we didn’t expect to see any sort of light-based signal from this merger, we could expect to see transient electromagnetic signatures in the case of a neutron star–black hole merger or a neutron star–neutron star merger — in the form of a kilonova or a short gamma-ray burst.
While we haven’t yet detected any mergers involving neutron stars, LIGO has the sensitivity to make these detections in the local universe, and we hope to start seeing them soon! Finding the electromagnetic companions to gravitational-wave signals would be the best way to probe the evolution history of the universe and learn what happens when evolved stars collide. So how do we hunt them down?
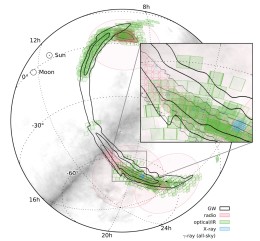
2D localization maps for LIGO’s detection of GW150914 (black contours), as well as the footprints of follow-up observations (red for radio, green for optical/IR, blue for X-ray). [Abbott et al. 2016]
Pinpointing a Volume
The two LIGO detectors can already provide rough 2D localization of where the gravitational-wave signal came from, but the region predicted for GW150914 still covered 600 square degrees, which is a pretty hefty patch of sky! In light of this, the simplest follow-up strategy of tiling large survey observations of the entire predicted region is somewhat impractical and time-consuming. Could we possibly take a more targeted approach?
The key, say a team of scientists led by Leo Singer (NASA Goddard SFC), is in using 3D estimates of the source location, rather than 2D sky maps: we need to produce distance estimates for the gravitational-wave source as well. Singer and collaborators have developed an algorithm that, from a gravitational-wave signal, can produce a fast full-volume estimate of the probability distribution for its source’s location.
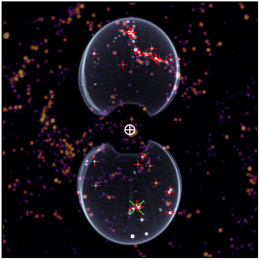
Volume rendering of the 90% credible region for a simulated gravitational-wave event, superimposed over a galaxy map for the region. Green crosshairs represent the true location of the source; the most massive galaxies inside the credible region are highlighted. Searching only these galaxies could significantly reduce the observing time needed to detect an electromagnetic counterpart. [Singer et al. 2016]
Targeted Efficiency
Singer and collaborators’ approach would make searching for electromagnetic counterparts to gravitational-wave events a much more efficient process. One particular advantage would be in reducing the number of false positives: for a typical wide-field follow-up campaign searching ~100 square degrees, hundreds of contaminating supernovae would be in the field. Targeting only 10’ x 10’ patches around 100 nearby galaxies, however, reduces the background to fewer than 10 contaminating supernovae.
An additional benefit is that this targeted strategy opens the door of gravitational-wave follow-up to many small-field-of-view, large-aperture telescopes, instead of limiting the task to broad synoptic surveys. This permits the involvement of many more campaigns in the hunt for the important electromagnetic counterparts to gravitational waves.
Note: Want to check out the team’s data? It’s publicly available here!
Citation
Leo P. Singer et al 2016 ApJL 829 L15. doi:10.3847/2041-8205/829/1/L15
1 Comment
Pingback: narrowing the FOV for LIGO detections