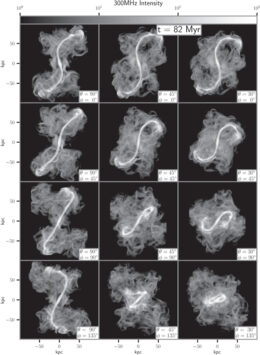
Simulated radio intensity of jets at various viewing angles. Click for high-resolution version. [Nolting et al. 2023]
At the centers of many galaxies, supermassive black holes accrete gas from their surroundings and shoot out a pair of immense jets of plasma. In some cases, these jets extend in opposite directions from the galaxy’s center, such as in the classic example of
Hercules A. Jets aren’t always so well behaved, though, and researchers have found examples of jets that snake and zigzag into S, X, and Z shapes. Using fluid dynamics simulations,
Chris Nolting (Los Alamos National Laboratory and College of Charleston) and collaborators explored possible causes of this alphabet soup of shapes, focusing on precession: slow changes in the axis of rotation of a spinning object. For the case of radio jets, precession could be caused by accretion instabilities or by a black hole companion to the central supermassive black hole. The simulation results, some of which are presented in the images above and to the right, show that a single jet configuration can appear vastly different when viewed from different angles. Unexpectedly, the team also found that jets can sometimes take sharp turns that can mimic the effects of outside forces, like shocks or winds.
Bonus
The team’s simulations are animated here, showing how the shapes of the radio jets change with time as well as with the viewing angle.
Citation
“Simulations of Precessing Jets and the Formation of X-shaped Radio Galaxies,” Chris Nolting et al 2023 ApJ 948 25. doi:10.3847/1538-4357/acc652