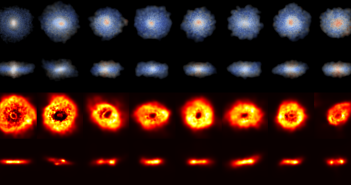
Forming Disk Galaxies Early in the Universe
What were galaxies like in the first 500 million years of the universe? According to simulations by Yu Feng (UC Berkeley) and collaborators, the earliest massive galaxies to form were mostly disk-shaped, rather than the compact clumps previously predicted.
Early-Galaxy Models
Current models for galaxy formation predict that small perturbations in the distribution of matter in the early universe collapsed to form very compact, irregular, clumpy first galaxies. Observations support this: the furthest out that we’ve spotted disk-shaped galaxies is at z=3, whereas the galaxies we’ve observed from earlier times — up to redshifts of z=8–10 — are very compact.
But could this be a selection effect, arising from the rarity of large galaxies in the early universe? Current surveys at high redshift have thus far only covered relatively small volumes of space, so it’s not necessarily surprising that we haven’t yet spotted any large disk galaxies. Similarly, numerical simulations of galaxy formation are limited in the size of the volume they can evolve, so resulting models of early galaxy formation also tend to favor compact clumpy galaxies over large disks.
An Enormous Simulation
Pushing at these limitations, Feng and his collaborators used the Blue Waters supercomputer to carry out an enormous cosmological hydrodynamic simulation called BlueTides. In this simulation, they track 700 billion particles as they evolve in a volume of 400 comoving Mpc/h — 40 times the volume of the largest previous simulation and 300 times the volume of the largest observational survey at these redshifts.
What they find is that by z=8, a whopping 70% of the most massive galaxies (over 7 billion solar masses each) were disk-shaped, though they are more compact, gas-rich, and turbulent than present-day disk galaxies like the Milky Way. The way the most massive galaxies formed in the simulation also wasn’t expected: rather than resulting from major mergers, they were built from smooth accretion onto the disks from nearby filaments.
These simulations suggest we still have a lot to learn about the structure of galaxies in the early universe and how they formed. Luckily, future telescope projects should help us out: Feng and collaborators estimate that the WFIRST satellite, for instance, should have the capability to detect 8000 disk galaxies of the type BlueTides predicts — compared to the weak 30% chance of finding a single one in the current largest-area Hubble survey!
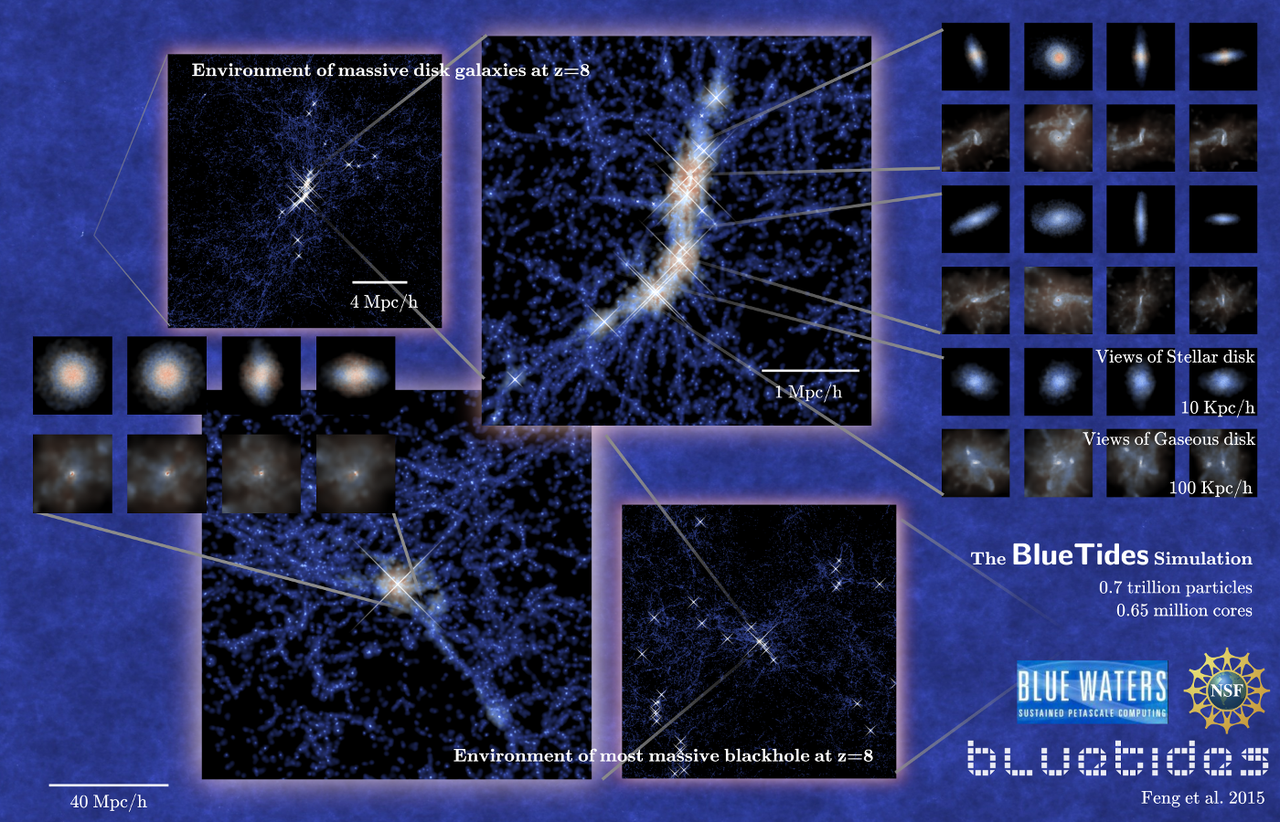
This graphic shows the scope of the BlueTides simulation; click to get a better look! The insets show the environment of the most massive black hole and the most massive disk galaxy at different scales. Crosses mark the positions of supermassive black holes. Credit: bluetides-project.org
Citation
Yu Feng et al. 2015 ApJ 808 L17. doi:10.1088/2041-8205/808/1/L17