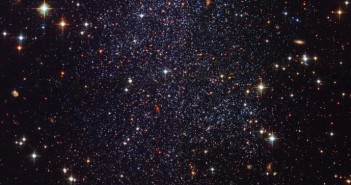
The Hunt for Dwarf Galaxies’ Ancestors
Dwarf galaxies are typically very faint, and are therefore hard to find. Given that, what are our chances of finding their distant ancestors, located billions of light-years away? A recent study aims to find out.
Ancient Counterparts
Dwarf galaxies are a hot topic right now, especially as we discover more and more of them nearby. Besides being great places to investigate a variety of astrophysical processes, local group dwarf galaxies are also representative of the most common type of galaxy in the universe. For many of these dwarf galaxies, their low masses and typically old stellar populations suggest that most of their stars were formed early in the universe’s history, and further star formation was suppressed when the universe was reionized at redshifts of z ~ 6–10. If this is true, most dwarf galaxies are essentially fossils: they’ve evolved little since that point.
To test this theory, we’d like to find counterparts to our local group dwarf galaxies at these higher redshifts of z = 6 or 7. But dwarf galaxies, since they don’t exhibit lots of active star formation, have very low surface brightnesses — making them very difficult to detect. What are the chances that current or future telescope sensitivities will allow us to detect these? That’s the question Anna Patej and Abraham Loeb, two theorists at Harvard University, have addressed in a recent study.
Entering a New Regime
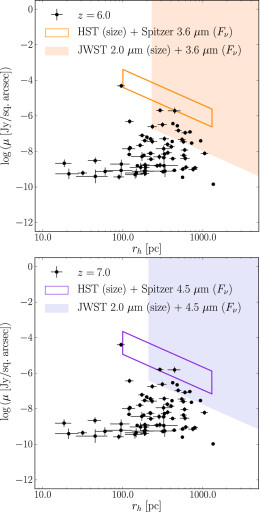
The surface brightness vs. size for 73 local dwarf galaxies scaled back to redshifts of z=6 (top) and z=7 (bottom). So far we’ve been able to observe high-redshift galaxies within the boxed region of the parameter space. JWST will open the shaded region of the parameter space, which includes some of the dwarf galaxies. [Patej & Loeb 2015]
Finally, the authors compared the properties of these 73 scaled-back dwarfs to those of high-redshift galaxies that we have already detected with the Hubble and Spitzer Space Telescopes, as well as to the detection limits of the upcoming James Webb Space Telescope (JWST) mission launching in 2018.
Patej and Loeb find that, when scaled back to redshifts of z = 6 or 7, the dwarf galaxies would be too faint to detect with current telescopes — despite being roughly the same size as high-redshift galaxies we’ve already detected. But the capabilities of JWST will push into this regime: according to Patej and Loeb’s calculations, JWST would be able to detect 13 of the 73 galaxies in the sample at a redshift of z = 6, and 9/73 at a redshift of z = 7.
Furthermore, the fraction of detectable galaxies would increase if these ancient dwarfs contained large numbers of Population-III-like, massive, bright stars. But even without such a boost, the hunt for the ancestors of local dwarf galaxies appears to be well within JWST’s capabilities!
Citation
Anna Patej and Abraham Loeb 2015 ApJ 815 L28. doi:10.1088/2041-8205/815/2/L28