Powerful solar flares are dazzlingly bright in ultraviolet and X-ray images of the Sun. Despite their demands for attention, there’s still a lot that we don’t know about these unpredictable eruptions.
Clues from 121.6 nm
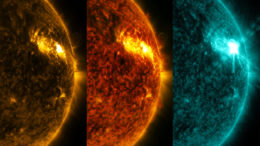
These Solar Dynamics Observatory images of the Sun show a solar flare in three extreme ultraviolet wavelengths. From left to right: 17.1, 30.4, and 13.1 nanometers. [NASA/GSFC/SDO]
To explore where flares get their energy, a team led by Jie Hong (Nanjing University, China) focused on a familiar feature of the ultraviolet solar spectrum: the 121.6-nm hydrogen Lyman-α emission line, produced by the roiling, turbulent hydrogen gas in the Sun’s atmosphere. The shape and behavior of the Lyman-α emission line can be used to learn about many different types of activity in the Sun’s chromosphere and corona — including solar flares.
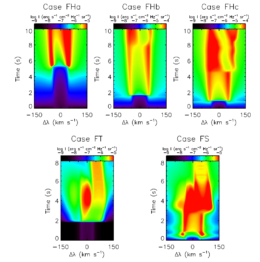
Evolution of Lyman-α profiles over time. The top row shows the time evolution of the profiles in the non-thermal heating case. The asymmetry of the peaks transitions from long to short wavelengths as time and energy increase. The bottom row shows the thermal heating case (left) and the thermal heating plus a soft electron beam case (right). In the thermal heating case, the double peak morphs into a single peak. Click to enlarge. [Hong et al. 2019]
Modeling Flare Emission
Hong and collaborators used radiative hydrodynamics to model solar flares heated by different mechanisms. Their goal was to explore how the type of heating might change the shape of the Lyman-α line we observe.
In particular, they examined two means of heating the flares: a thermal mechanism where the energy comes from conduction from nearby plasma, and a non-thermal mechanism where the heat is provided by a beam of energetic electrons generated by magnetic reconnection. In the non-thermal case, they also varied the strength of the heating by an order of magnitude.
After allowing the modeled flares to evolve for eight or ten seconds, the researchers looked for subtle changes in the shape of the Lyman-α profile that could be linked to the underlying heating mechanism.
The asymmetries and peaks of the modeled emission lines showed distinctive patterns and behavior over time — fingerprints, Hong and collaborators argue, that could help identify the source of heat for an observed flare.
Flare Photography
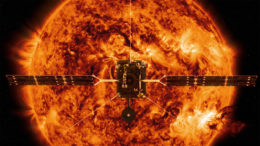
An artist’s impression of Solar Orbiter silhouetted against the Sun. The spacecraft’s tilted orbit will provide never-before-seen images of the Sun’s poles. [Spacecraft: ESA/ATG medialab; Sun: NASA/SDO/P. Testa (CfA)]
In particular, they look forward to the joint NASA-ESA Solar Orbiter mission, set to launch in 2020, which will be the first spacecraft to snap extreme-ultraviolet pictures of the Sun from out of the ecliptic plane, and China’s Advanced Space-Based Solar Observatory (ASO-S), which is scheduled for launch in 2022. ASO-S will carry a dedicated Lyman-α imager.
After decades of observations, it looks like the field of flare research is still heating up!
Citation
“The Response of the Lyα Line in Different Flare Heating Models,” Jie Hong et al 2019 ApJ 879 128. doi:10.3847/1538-4357/ab262e