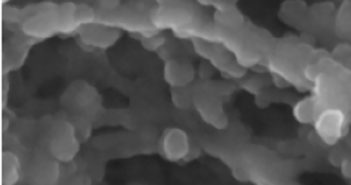
Featured Image: Making Molecules on Dust
How do molecules form in the hostile environments of the circumstellar and interstellar medium? Laboratory experiments are helping us to better understand this process! The image above (click for the full view) shows a high-resolution electron microscopy image of the complex surfaces of silicate grains, representative of dust grains found in the space around and between stars. In a new study led by Alexey Potapov (Max Planck Institute for Astronomy, Germany), scientists deposited thin layers of carbon dioxide and ammonia ices on nanometer-sized silicate and carbon grains and explored the subsequent chemical reactions under conditions similar to those of the interstellar medium. In the team’s experiments, the reactions that form complex organic molecules happened three times as fast in the ice on the surface of dust grains as they did in the ice alone. This catalytic effect of dust surfaces is an important piece in the puzzle of how complex molecules — and ultimately, life — formed in our universe. You can read the full article below to find out more!
Citation
“Evidence of Surface Catalytic Effect on Cosmic Dust Grain Analogs: The Ammonia and Carbon Dioxide Surface Reaction,” Alexey Potapov et al 2019 ApJL 878 L20. doi:10.3847/2041-8213/ab2538