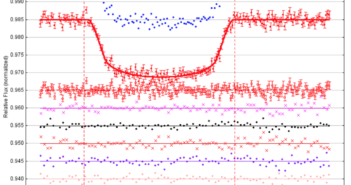
Selections from 2017: Image Processing with AstroImageJ
Editor’s note: In these last two weeks of 2017, we’ll be looking at a few selections that we haven’t yet discussed on AAS Nova from among the most-downloaded papers published in AAS journals this year. The usual posting schedule will resume in January.
AstroImageJ: Image Processing and Photometric Extraction for Ultra-Precise Astronomical Light Curves
Published January 2017
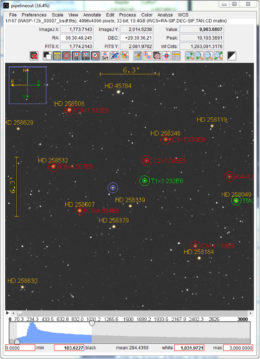
The AIJ image display. A wide range of astronomy specific image display options and image analysis tools are available from the menus, quick access icons, and interactive histogram. [Collins et al. 2017]
Main takeaway:
AstroImageJ is a new integrated software package presented in a publication led by Karen Collins (Vanderbilt University, Fisk University, and University of Louisville). It enables new users — even at the level of undergraduate student, high school student, or amateur astronomer — to quickly start processing, modeling, and plotting astronomical image data.
Why it’s interesting:
Science doesn’t just happen the moment a telescope captures a picture of a distant object. Instead, astronomical images must first be carefully processed to clean up the data, and this data must then be systematically analyzed to learn about the objects within it. AstroImageJ — as a GUI-driven, easily installed, public-domain tool — is a uniquely accessible tool for this processing and analysis, allowing even non-specialist users to explore and visualize astronomical data.
Some features of AstroImageJ:
- Image calibration: generate master flat, dark, and bias frames
- Image arithmetic: combine images via subtraction, addition, division, multiplication, etc.
- Stack editing: easily perform operations on a series of images
- Image stabilization and image alignment features
- Precise coordinate converters: calculate Heliocentric and Barycentric Julian Dates
- WCS coordinates: determine precisely where a telescope was pointed for an image by PlateSolving using Astronomy.net
- Macro and plugin support: write your own macros
- Multi-aperture photometry with interactive light curve fitting: plot light curves of a star in real time
Citation
Karen A. Collins et al 2017 AJ 153 77. doi:10.3847/1538-3881/153/2/77