Editor’s note: We’re wrapping up a busy summer with one last conference: the EPSC-DPS joint meeting in Geneva, Switzerland. To celebrate the announcement of AAS Publishing’s new Planetary Science Journal, we’ll be bringing you some highlights from this planetary science conference all week!
DPS Kuiper Prize Lecture: Inside the Moon Fifty Years After Apollo
The DPS’s 2019 Gerard P. Kuiper Prize for outstanding contributions to the field of planetary science has been awarded to Maria Zuber (MIT) “for her contributions to advancements in geophysics, planetary gravity mapping, and laser altimetry.”
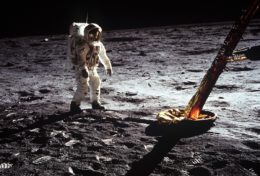
Apollo 11 astronaut Buzz Aldrin stands on (and doesn’t sink into!) the surface of the Moon. [NASA]
Zuber opened her prize lecture by pointing out how much we didn’t know about the Moon before the first lunar landing fifty years ago. We weren’t sure what to expect regarding the Moon’s structure and composition, nor how that could influence the landing — was the lunar dust so soft and thick that it would swallow the lander like quicksand? Fortunately, none of our uncertainties about the Moon derailed the landing — and with research since then, we now much better understand the lunar crust and interior.
An important means by which we’ve learned about the Moon’s structure is the Gravity Recovery and Interior Laboratory (GRAIL) mission, led by Zuber, which lasted from 2011 to 2012. By flying two spacecraft close over the Moon’s surface and measuring minute changes in the distance between them, the GRAIL team was able to construct an extremely detailed map of the Moon’s gravitational field, thereby revealing its internal geological structure.
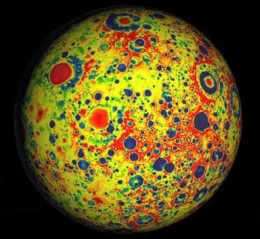
Variations in the Moon’s gravitational field, as measured by GRAIL. [NASA/JPL-Caltech/MIT/GSFC]
GRAIL’s data produced many important results, including detailed maps of the lunar crust and lithosphere, insight into the subsurface structure of the Moon’s 74 impact basins with diameters greater than 200 km, and constraints on the presence of a core at the Moon’s center. One of the biggest surprises from the mission, says Zuber, was the discovery of the Moon’s fractured crust: even beneath the lunar surface, the Moon’s crust is broken up. GRAIL’s insights provide a record of massive impacts in the inner solar system early in its history, as well as a look at some of the formation processes that were at work during the Moon’s birth.
Could a mission like GRAIL be applied to other solar-system bodies in the future? In order to build its detailed maps, GRAIL dropped to within a handful of kilometers of the lunar surface — a feat that wouldn’t be possible around any of our solar system’s planets. If we tried that with a spacecraft around Mercury, for instance, the thermal radiation would destroy the spacecraft.
But perhaps a mission like GRAIL could be used to map other satellites in our solar system, such as Jupiter’s Galilean moons? Fifty years after we first landed on the Moon, it’s exciting to see how much we’ve learned since then — as well as how much we still stand to learn, both about Earth’s only natural satellite and about other, similar bodies in our solar system.
DPS Urey Prize Lecture: New Horizons: Exploration of Distant Worlds in the Kuiper Belt
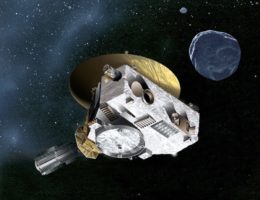
Artist’s illustration of the New Horizons spacecraft flying by a Kuiper-belt object. [NASA/JHUAPL/SWRI]
In a transition from nearby bodies, our next speaker took us to the outer reaches of the solar system with the New Horizons mission.
Kelsi Singer (Southwest Research Institute), this year’s DPS Urey Prize winner for outstanding achievement in planetary research by a young scientist, gave us an update on this intrepid little explorer’s latest adventures.
New Horizons launched in January 2006, and after a gravity assist from Jupiter, it arrived at the Pluto system in July of 2015. After flying by Pluto and taking detailed images of the former planet and its moon Charon, New Horizons then continued onward, later buzzing the Kuiper belt object 2014 MU69 in January of this year.
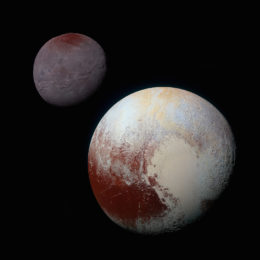
This composite image with enhanced colors shows New Horizons observations of Pluto (foreground) and Charon (background). These Kuiper belt bodies, as well as MU69, appear to have very few craters. [NASA/JHUAPL/SwRI]
New Horizons’s images have been nothing short of spectacular (see, for instance, the incredible detail in the image of Pluto that’s on
The Planetary Science Journal‘s cover and shown at the top of the page. No seriously,
check out the high-res version. Zoom in. It’s unreal!). Singer showed us some of the images of Charon that reveal a whole host of features: a mountain surrounded by a moat, ropey structures from icy volcanic eruptions, wide flat plains. All of these features are clues that can be used to piece together Charon’s past — in this case indicating that the moon was resurfaced long ago, and it has been sitting gathering crater impacts since.
The craters, however, raise another new puzzle. Singer highlighted the lack of small (< 10 km in diameter) craters relative to what we expected to see, and showed the same missing-crater trend in New Horizons’s images of MU69. As these craters should have been caused by Kuiper belt objects of < 1 km in size, their dearth suggests there may be far fewer small Kuiper belt objects than we thought. This suggests one of the following:
- Fewer small objects tend to form in the Kuiper belt than we initially thought, or
- Small objects do form, but they somehow get preferentially removed.
Either explanation would have important implications for how our solar system formed and evolved, and more observations like those of New Horizons will prove invaluable in puzzling out this mystery!
EPSC Farinella Prize Lectures: Completing the Inventory of the Solar System
The 2019 Farinella prize was awarded for the first time to a team of people rather than to a single individual. Scott Sheppard (Carnegie Institution for Science) and Chad Trujillo (Northern Arizona University) jointly received the award for their collaborative work observationally characterizing the Kuiper belt and Neptune-trojan population.
Neptune Trojans
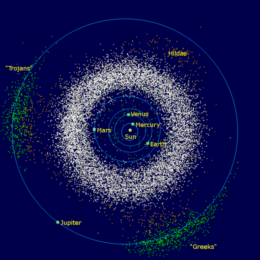
Diagram showing the locations of the two groups of Jupiter trojans (seen in green; the ones that trail Jupiter’s orbit are called “Trojans” and the ones that lead Jupiter’s orbit are called “Greeks”). [Mdf]
What are trojans? These small celestial bodies (mostly asteroids) orbit the Sun along the same path as the planet with which they’re associated, but they either lead 60° ahead or trail 60° behind the planet at so-called
stable Lagrange points. The Jovian trojans are perhaps the most well known — there are more than 7,000 known asteroids that move in these two clumps ahead of and behind Jupiter. But other planets have trojans as well: we’ve spotted four around Mars, a pair around Uranus, and even one around Earth.
Neptune, at current count, has 23 known — and Sheppard and Trujillo have discovered a number of them. When the duo first started looking for Neptune trojans, it was unclear whether the majority of these bodies would be on high- or low-inclination orbits. Sheppard and Trujillo’s work, however, suggests that the vast majority of Neptune trojans are high-inclination (high-inclination orbits dominate by a ratio of 4 to 1!). This puffed-up population points to an interesting origin: they were likely captured by Neptune from elsewhere in the solar system during a period of migration for the giant planets.
As Neptune trojans share a lot of similar properties with Jupiter trojans, we can guess that these two populations may have similar histories. With any luck, we’ll be able to test this theory soon: in 2021, NASA plans to launch a space probe called Lucy to visit six different Jupiter trojans. This mission is sure to reveal more about these intriguing bodies.
Extreme TNOs
What other unexpected objects lurk deep in the outer reaches of our solar system? The minor planet Sedna, which lies at a distance of about 86 AU (three times the distance to Neptune), poses an interesting riddle with its extremely eccentric orbit. With a number of plausible formation scenarios proposed — each with different implications for the solar system’s formation environment and subsequent evolution — Sheppard and Trujillo determined that the best way to understand Sedna was to find more bodies like it!
Thus began an extensive deep solar system survey, using the CTIO 4-m telescope with the Dark Energy Camera in the southern hemisphere and the Subaru 8-m telescope with HyperSuprimeCam in the northern hemisphere. This large uniform survey has covered 3,000 square degrees, or about 20% of the sky, to 24th magnitude — and through this search, Sheppard and Trujillo have discovered roughly 80% of the known objects beyond 60 AU.
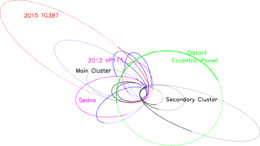
Schematic showing the alignment of the orbits of detached eTNOs and the proposed orbit of a hypothetical super-Earth-mass planet (in green). A secondary cluster of aligned bodies is also predicted in this model, and is observed. [Sheppard et al. 2019]
The survey revealed two Sedna-like objects — 2012 VP
113, a distant minor planet with a whopping perihelion of ~80 AU; and “The Goblin”, 2015 TG
387, which has an orbital period of more than 11,000 years — as well as a number of additional extreme trans-Neptunian objects (eTNOs), bodies with perihelions of >40 AU and semimajor axes of >150 AU. Intriguingly, a pattern appeared in the data: all of the orbits of the eTNOs appeared to cluster in approximate alignment, rather than being randomly distributed around the Sun.
Could a distant, massive, as-yet undiscovered planet in our solar system be influencing these bodies’ orbits, shepherding them into a cluster? That’s what Sheppard and Trujillo proposed, and this theory has been supported by additional eTNOs that have been found since.
The distant-object discoveries continue to pour in — two of the latest are FarOut (2018 VG18), which currently lies at ~120 AU and FarFarOut (no designation yet), which is estimated to be at a whopping near-140-AU distance! These continued discoveries bring us ever closer to understanding our outer solar system — and possibly finding any hidden planets that may lurk there.
Splinter Session: Status Report on Planning for the Next Planetary Science Decadal Survey
What’s planned for the next decade of planetary science? That’s exactly what needs to be decided via the next Planetary Science Decadal Survey, a review process in which the community comes together to define and prioritize the key scientific questions that could potentially be addressed in the next decade, as well as prioritize the possible missions that might address these questions.
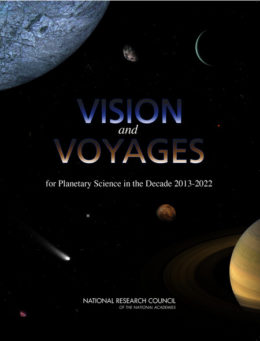
Cover for the last planetary science decadal survey. [National Research Council for the National Academies]
You may have thought we just went through this whole process recently — but it turns out planetary science has its own overview, which occurs completely independently of the astronomy decadal survey (
Astro 2020 is currently underway). The planetary science decadal is offset by a few years, so the field is only just starting to gear up for this review.
In this session, David Smith (Space Studies Board, NAS Engineering and Medicine), Lori Glaze (director of NASA’s Science Mission Directorate’s Planetary Science Division), and Linda French (program director at NSF) were on hand to discuss plans and processes for the upcoming planetary science decadal. The estimated timeline, as it currently stands:
- 2/2020: Opening of the website through which scientists can submit white papers, short papers that describe important science questions, missions, or the state of the field
- 5/2020: White paper submission deadline
- 6/2020: The decadal survey committee and panels, made up of members of the planetary science community, begin meetings to consider the white papers and mission studies
- 10/2021: The survey committee compiles their recommendations into the first draft of a report with recommendations for the coming decade
- 3/2022: The final survey report is released
Keep an eye out next year for the start of this important process!