From spacecraft that dive into the Sun’s atmosphere to insights from complex models, we’re learning more about the Sun than ever before. Today’s highlight introduces five recent research articles that tackle hot topics in solar physics.
Tracking Down Dark Regions in the Corona
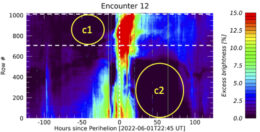
An example of two darkened regions (circled) of the corona seen by Parker Solar Probe. The vertical dashed line indicates the moment when the spacecraft passed closest to the Sun, at a distance of just 13.29 solar radii (5.7 million miles or 9.2 million kilometers). Click to enlarge. [Adapted from Stenborg et al. 2023]
To investigate further, Stenborg and coauthors compared the locations of the darkened regions to the locations of coronal holes — places in the corona where the solar magnetic field extends out into the solar system rather than looping back to the solar surface, allowing particles to stream out from the Sun. The team found that many of the darkened regions can be explained as the spacecraft’s line of sight passing through the “zone of influence” of a coronal hole. Other darkened regions had a different cause: as coronal mass ejections exit the corona, they leave a plasma-depleted region in their wake. The largest coronal mass ejection witnessed by the spacecraft excavated both plasma and dust.
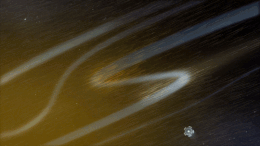
An animation showing the Parker Solar Probe traversing a solar switchback. [NASA’s Goddard Space Flight Center/Conceptual Image Lab/Adriana Manrique Gutierrez]
Seeking the Source of Switchbacks
When the Parker Solar Probe made its first journeys toward the Sun, it discovered rapid back-and-forth changes in the direction of the solar wind magnetic field that researchers dubbed switchbacks. There are many theories as to the origins of solar switchbacks, including magnetic field lines rearranging into a new configuration (i.e., magnetic reconnection), turbulence in the solar wind, or a combination of both.
To delve into the origins of switchbacks, Pankaj Kumar (American University and NASA Goddard Space Flight Center) and collaborators investigated a closely associated phenomenon called microstreams. Microstreams are changes in the speed and direction of the solar wind that last about 10 hours and seem to be linked to switchbacks. Using data from the Parker Solar Probe as it surfed the solar wind, and combining it with photographs taken from afar by the Solar Dynamics Observatory, Kumar’s team found that microstreams in the solar wind and jets produced deeper in the solar atmosphere vary over time in the same way. The jets are also associated with close-set opposing magnetic field lines and bursts of protons and ions — clear signs that magnetic reconnection is underway. This suggests that magnetic reconnection creates the jets, and the jets in turn create switchbacks and microstreams.
Improving Forecasts with Machine Learning
Correctly forecasting the arrival times of coronal mass ejections — immense explosions of tangled solar plasma and magnetic fields — at Earth is important for testing our understanding of how these eruptions travel through space as well as for developing an early warning system. Even as simulations of coronal mass ejections unfurling across space have grown more complex, however, the typical error in the arrival times they predict has remained the same, around 12 hours.
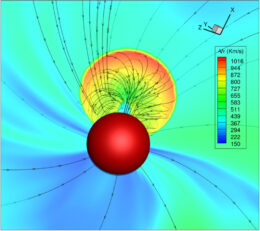
Example of a modeled coronal mass ejection before it erupts into interplanetary space. [Singh et al. 2023]
Solving the Cool Chromosphere Problem
Samuel Evans (Boston University) and collaborators focused on the chromosphere, the region between the sunspot-dotted solar surface and the tenuous, superheated corona. The chromosphere represents a unique challenge for modelers because one of our best tools — fluid dynamics models — can’t quite capture what’s going on there; the corona, where fluid dynamics models excel, is so rarefied that different components of the plasma can be treated individually, as they rarely interact. The chromosphere, however, is dense enough that the interactions between different components of the plasma can no longer be ignored. So far, fluid dynamics models have struggled to reproduce the temperature in the solar chromosphere — likely somewhere in the 3000–4000K range, based on observations — predicting a relatively chilly 2000K.
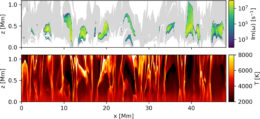
Top: Areas where the instability is at work. Bottom: Simulated chromospheric temperature. The instability grows fastest where the chromosphere is coolest. Click to enlarge. [Evans et al. 2023]
Gaining X-ray Insights into Charged-Particle Acceleration
Solar flares are likely the most well-known solar phenomenon, but there’s still plenty we don’t know about them. We know that solar flares accelerate charged particles to high velocities by releasing pent-up magnetic energy, but the details of this process are fuzzy.
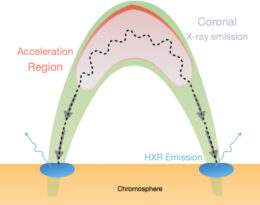
Cartoon demonstrating the location of the particle acceleration region and the source locations for X-ray emission and hard X-ray (HXR) emission. Click to enlarge. [Adapted from Stores et al. 2023]
Citation
“Investigating Coronal Holes and CMEs as Sources of Brightness Depletion Detected in PSP/WISPR Images,” Guillermo Stenborg et al 2023 ApJ 949 61. doi:10.3847/1538-4357/acd2cf
“New Evidence on the Origin of Solar Wind Microstreams/Switchbacks,” Pankaj Kumar et al 2023 ApJL 951 L15. doi:10.3847/2041-8213/acd54e
“Improving the Arrival Time Estimates of Coronal Mass Ejections by Using Magnetohydrodynamic Ensemble Modeling, Heliospheric Imager Data, and Machine Learning,” Talwinder Singh et al 2023 ApJ 948 78. doi:10.3847/1538-4357/acc10a
“Multifluid Simulation of Solar Chromospheric Turbulence and Heating Due to Thermal Farley–Buneman Instability,” Samuel Evans et al 2023 ApJ 949 59. doi:10.3847/1538-4357/acc5e5
“Spectral and Imaging Diagnostics of Spatially-Extended Turbulent Electron Acceleration and Transport in Solar Flares,” Morgan Stores et al 2023 ApJ 946 53. doi:10.3847/1538-4357/acb7dc