Editor’s Note: This week we’ll be writing updates on selected events at the 54th Division for Planetary Sciences meeting in London, Ontario, and online. This post covers the last three days of the meeting. The usual posting schedule for AAS Nova will resume on October 10th.
Table of Contents:
- Bold Ideas Plenary Session
- Planetary Atmospheres and the Search for Signs of Life Beyond Earth (Sara Seager)
- A Unique Origins Survey: Gaia-Enabled Occultation Measurements of Small Bodies in the Outer Solar System (Marc Buie)
- Astrobiology in the Era of Big Data: Leveraging Chemometrics for Prebiotic Chemistry and Planetary Exploration (Laura Rodriguez)
- Workforce Plenary Session
- Press Conference
- Looking Forward/Looking Back Plenary Session
- Questions Convey Us: Our Human Exploration of the Solar System, 1962 to Now (and After) (Emily Lakdawalla)
- The James Webb Space Telescope: A New Era of Science for All (Eric Smith)
- A Future Mission to Uranus: Exploration of Five Possible Ocean Worlds and a Bevy of Small Icy Moons (Richard Cartwright)
Bold Ideas Plenary Session
Planetary Atmospheres and the Search for Signs of Life Beyond Earth (Sara Seager)
Just 30 years ago, exoplanets were a wild and unpromising idea. Even when the first transiting exoplanet was discovered in 1999, many researchers thought the field wouldn’t go anywhere; sure, exoplanets exist, but the measurements are just too hard to make. In the first of today’s plenary talks, Sara Seager (Massachusetts Institute of Technology) reminded the audience that the line between what is accepted and what is dismissed is constantly changing, and ideas that seem outlandish may someday advance our understanding.
Seager’s talk focused on the search for life on worlds outside our solar system, especially the concept of biosignatures: chemical compounds in the atmosphere of a planet that indicate that life is present. While detecting life in this way sounds simple, Seager delineated the many reasons that it’s not. Part of the issue is our observational constraints, which push us to search for life-bearing planets around the smallest stars, M dwarfs, in search of a strong signal. M dwarfs are far more active and variable than the Sun, which spells trouble for the observational side of the issue (variability can drown out subtle atmospheric signals) as well as the biological side — during an M dwarf’s lengthy “teenage” phase, its extreme thermal output might doom any life that arises on nearby planets.
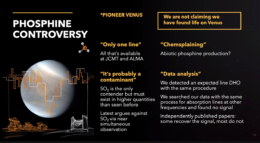
An explanation of the main objections to the discovery of phosphine in Venus’s atmosphere. Click to enlarge. [Slide by Sara Seager]
This process shows that science is working, Seager notes, and the back and forth incited by the potential discovery of a biosignature gas in our planetary backyard will likely play out for years to come. And we know far more about Venus than we do about any exoplanet — imagine the debate that would follow the first detection of phosphine on an exoplanet! (As an aside, Seager noted that phosphine is hardly the most outlandish Venus-related proposal — major depletion of sulfur dioxide in Venus’s atmosphere could be explained by ammonia-producing life that partially neutralizes the sulfuric acid within the clouds. A tidy explanation, or optimistic humans connecting unrelated data points? It’s up for debate!)
Seager concluded with a bevvy of tantalizing (or unpromising, depending on your opinion!) possibilities: Breakthrough Starshot would send thousands of miniature spacecraft on a life-finding mission; a solar gravitational lens telescope would boost our technological capabilities; and an Earth-like planet orbiting a white dwarf would give us a chance to detect gases associated with technological processes, like sulfur hexafluoride. The possibilities are wild and endless — buckle up for a decades-long search for life beyond Earth!
A Unique Origins Survey: Gaia-Enabled Occultation Measurements of Small Bodies in the Outer Solar System (Marc Buie)
In order to understand the origins of our solar system, we need to understand the small bodies of the outer solar system. So says Marc Buie (Southwest Research Institute), who chases outer solar system bodies from Earth by watching them block the light from distant stars. Part of the challenge of studying these far-flung objects is that you need to study a lot of them to make firm conclusions, so chasing down individual objects with an expensive spacecraft, à la New Horizons’s pursuit of Pluto and Kuiper belt object Arrokoth, is infeasible. Instead, ground-based efforts with telescopes of modest size can play an important role.
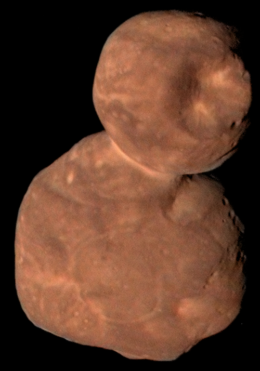
A composite image of the Kuiper belt object Arrokoth, which is a contact binary. [NASA/Johns Hopkins University Applied Physics Laboratory/Southwest Research Institute/Roman Tkachenko]
Using data from the Gaia spacecraft, which has made exceptionally precise measurements of the positions and motions of stars in the Milky Way, Buie anticipates being able to study large numbers of outer solar system objects using stellar occultations. This technique has already allowed us to detect 10-km-scale surface features on the five asteroid targets of the Lucy mission, and it should allow us to constrain collisional processing and cratering of outer solar system objects without sending a spacecraft to them. Multiple possibilities exist for implementing this kind of program, including a fixed robotic array of thirty 28-cm telescopes or a mobile array of small telescopes transported worldwide by trained observers.
While the price tag for implementation might seem large — Buie estimates that observing ten 20-km Kuiper belt objects would require $7 million to cover equipment, travel, and personnel costs — it’s far cheaper than a spacecraft mission. Buie points out that rather than a scientific or technological stumbling block, the issue is a programmatic one; while funding exists for small, speculative projects and massive, spaceflight-ready endeavors, there is something of a funding desert in between these two extremes, making what Buie calls “team science” difficult to get started. In the future, Buie hopes that the planetary science community will consider the kinds of team science it wants to pursue in addition to the kinds of spacecraft missions it hopes to launch.
Astrobiology in the Era of Big Data: Leveraging Chemometrics for Prebiotic Chemistry and Planetary Exploration (Laura Rodriguez)
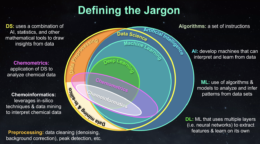
An explanation of where chemometrics fits in relative to other data science and artificial intelligence concepts. Click to enlarge. [Slide by Laura Rodriguez]
Prebiotic chemistry is often studied as a way to probe the origins of life on Earth, but it’s also important for predicting where else in the solar system we might find life. By delving into the formation of molecules in different sites around the solar system, we can start to understand the conditions necessary for life to evolve as well as evaluate compounds proposed as biosignatures — even large molecules like ATP (an important organic molecule in the human body that helps deliver energy to our cells) can form without life being present.
Planetary science missions, especially those tasked with searching for life, represent the intersection of the study of prebiotic chemistry and machine learning. Spacecraft missions have reached the point where the instruments are able to collect a massive amount of complex data. While it’s possible for a spacecraft to dutifully beam these data back to Earth to be pored over by scientists, this process is slow and energy consuming, especially for spacecraft exploring the icy worlds of the outer solar system. Instead, Rodriguez proposes that spacecraft be given the tools to make the first analysis pass themselves, engaging in preprocessing steps that help them decide what to do next, without human intervention.
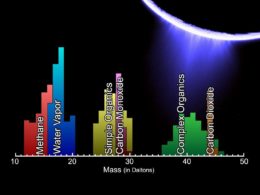
A simplified mass spectrum showing the types of compounds detected in the plumes of Enceladus by the Cassini spacecraft. Click to enlarge. [NASA/JPL/SwRI]
Ultimately, chemometrics will benefit many planned and proposed missions such as the Mars Sample Return program, a Europa lander, and a Ceres sample return mission, allowing spacecraft to make the decisions necessary to optimize limited time, energy, and resources.
Workforce Plenary Session
Behind the Myth of Meritocracy: How STEM Fields Perpetuate Racial and Gender Disparities (Adia Harvey Wingfield)
Adia Wingfield, a professor of sociology from Washington University in St. Louis, described the current state of racial and gender diversity in science, technology, engineering, and math (STEM) fields in the United States, the issues facing scientists and engineers belonging to underrepresented groups, and how organizations can work to improve the representation of these groups.
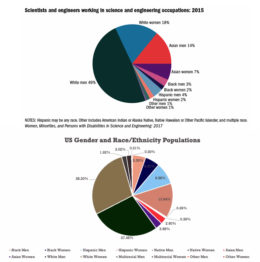
Comparison of the demographics of the STEM workforce (top) to the demographics of the US population (bottom). Click to enlarge. [Adapted from slides by Adia Wingfield]
What’s the cause of this disparity? There are several common — and incorrect — assumptions about the causes of workplace disparities: women tend to prioritize family over work; Black and Latino workers aren’t as qualified; it’s hard to recruit these workers, and once they’re recruited they leave for other opportunities; and STEM is a meritocracy where everything is fair and equal, so these disparities are really out of our control.
In reality, there are biases in the hiring process that disproportionately impact workers belonging to underrepresented groups; unspoken norms and organizational culture can create a “chilly” environment for many employees; harassment is pervasive; and tokenism remains an issue. These issues are wide ranging, and some issues affect people belonging to certain groups more than others (e.g., gender and sexual minorities are more likely to face issues with sexual harassment; tokenism is a complex issue, especially for people who are in the majority with respect to gender but in the minority with respect to race).
As academics, we can learn from the techniques used in corporate settings. Targeted recruitment — turning to historically Black colleges and universities and predominantly Latino-serving institutions rather than solely leveraging existing networks — can open up organizations to job candidates who would otherwise not be part of the applicant pool; formal mentoring programs help connect new hires belonging to underrepresented groups to the rest of the organization, and these programs are especially successful when mentors are incentivized to be invested in the success of their mentees; sexual harassment training with a helpful, rather than punitive, focus (e.g., focusing on bystander intervention rather than the legal consequences of harassment) can improve the organizational climate; and diversity task forces that are given the power to enact change and involve people from all levels of an organization can increase the proportion of historically excluded groups at the managerial level. These techniques, which in a five-year survey were shown to increase recruitment and retention of minoritized individuals, are likely applicable to academic institutions as well.
Press Conference
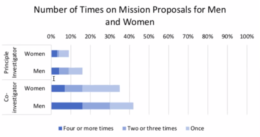
Survey results regarding participation in mission proposals as principal investigator and co-investigator. [From slide by Julie Rathbun]
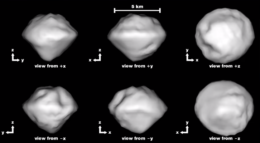
A shape model of asteroid 3200 Phaethon derived from stellar occultation observations. [From slide by Sean Marshall]
You can watch a recording of this press conference on the AAS Press YouTube channel.
Looking Forward/Looking Back Plenary Session
Questions Convey Us: Our Human Exploration of the Solar System, 1962 to Now (and After) (Emily Lakdawalla)
Freelance science writer and planetary scientist Emily Lakdawalla kicked off the final plenary of DPS 54 with a perspective on the history and future of planetary science. Planetary science was born of the merger of two scientific endeavors: astronomy and geography. Astronomy began with humans peering at the night sky, trying to understand what importance the stars held for us, and asking questions about our origins. Initially a solitary endeavor, early astronomy was limited to those with the money to build expensive telescopes. And while early astronomers sometimes guarded their data jealously, astronomy has evolved into one of the most open of the scientific fields.
Geography, on the other hand, began with large surveys funded by the reigning governments of newly conquered territories. These surveys thus involved large numbers of people working together, and they evolved to incorporate information about natural hazards such as flood or earthquake risks, which was shared publicly in accessible databases. It’s impossible to ignore the checkered history of geography, which played an important role in assessing the value of lands acquired forcefully in the colonial era.
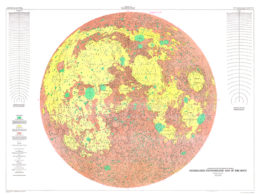
The first map of the Moon created by the US Geological Survey. [USGS]
Lakdawalla commented on the traditions — both positive and negative — that planetary science retains from its forebears, astronomy and geography. The secretive guarding of data remains an issue (Lakdawalla recounted the case of a spacecraft instrument whose calibration method was never written down in order to maintain the relevance of the sole expert who knew the method; those data are now nearly useless), but planetary science has evolved into an incredibly open field; the existence of the arXiv and the Planetary Data System are proof of that fact.
Lakdawalla closed with some notes of caution for our field: as our exploration of the solar system continues, we must be mindful not to follow the exploitative origins of geography as we consider how best to study these worlds without contaminating them; we must be patient when planning ambitious, decades-spanning missions, and be mindful that these missions may not be for us, but for others who will benefit from them in the future; we’ll need to work hard to convey the importance of the moons and small bodies in our solar system now that all of the planets have been visited by at least one spacecraft; and we should seek to combat the colonialistic tendencies of private space exploration by being sure to include everyone in space exploration, especially those who have been traditionally excluded.
The James Webb Space Telescope: A New Era of Science for All (Eric Smith)
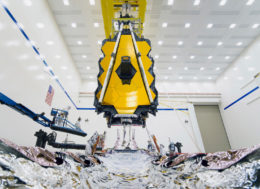
JWST, fully assembled for the first time. [NASA/Chris Gunn]
Smith noted that planetary science proposals during Cycle 1 were particularly successful, with the proportion of selected planetary science proposals being larger than the proportion of submitted proposals. Solar system science will use 7% of the observing time during Cycle 1, amounting to 607.5 hours, 25% of which has already been completed. More than a third of the Cycle 1 planetary science proposals have no exclusive use period, which means the data will become publicly available immediately. Exoplanet science makes up 29% of Cycle 1, requiring 2,516.7 observing hours for 96 programs. Smith commented that several of the Cycle 1 proposals were actually archival proposals, perhaps making this the first mission for which archival proposals were selected for data that hadn’t yet been taken!
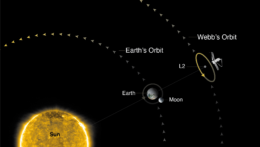
Illustration of JWST’s berth at L2. [STScI]
A Future Mission to Uranus: Exploration of Five Possible Ocean Worlds and a Bevy of Small Icy Moons (Richard Cartwright)
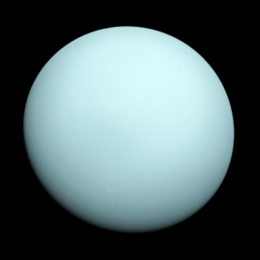
A classic Voyager 2 photo of Uranus, taken in 1986. [NASA/JPL-Caltech]
Luckily, the most recent Planetary Decadal Survey prioritized an orbiter and probe to Uranus, opening an avenue for us to answer these questions. Cartwright gave an overview of what we know about Uranus’s moons, what we don’t, and how we might design a spacecraft to the Uranian system to get answers.
Uranus’s 13 ring moons form the most compact group of satellites in our solar system. Only Puck has been spatially resolved in previous observations, and we don’t know the ages of these objects or what their surfaces are like. Perhaps most intriguing of the ring moons is the outermost, Mab, which is likely only 6–12 km in diameter and is probably associated with the dusty μ ring. Given the compactness of the system, it’s likely that the moons share material in an interesting way.
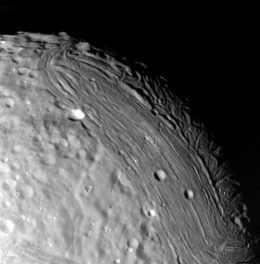
A detailed view of the surface of Uranus’s moon Miranda. [JPL]
In terms of a potential future mission to the Uranian system, Cartwright recommends an orbiter, which offers the best chance of studying internal oceans and geologic processes on the moons. An ideal suite of instruments would include a magnetometer, cameras (optical and mid-infrared), a near-infrared mapping spectrometer, particle and dust detectors, and an ultraviolet spectrograph. Timing is important, too — launching the mission in time to catch the Jupiter gravity assist window in 2030–2034 would get us to Uranus faster and get this long-awaited science started!