Editor’s Note: This week we’re at the 243rd AAS meeting in New Orleans, LA. Along with a team of authors from Astrobites, we will be writing updates on selected events at the meeting and posting each day. Follow along here or at astrobites.com for daily summaries, or follow @astrobites.bsky.social on BlueSky for more coverage. The usual posting schedule for AAS Nova will resume on January 16th.
Table of Contents:
- Plenary Lecture: Radio Astrophysics and Cosmology from the Moon, Jack Burns (University of Colorado, Boulder)
- Press Conference: Exoplanets and Brown Dwarfs
- High Energy Astrophysics Division Bruno Rossi Prize Lecture: Anatoly Spitkovsky (Princeton University)
- Press Conference: High-Energy Phenomena and Their Origins
- Annie Jump Cannon Prize Lecture: Theories of Planet Formation, Eve Lee (McGill University)
- Plenary Lecture: Sukanya Chakrabarti (University of Alabama, Huntsville)
Plenary Lecture: Radio Astrophysics and Cosmology from the Moon, Jack Burns (University of Colorado, Boulder) (by Briley Lewis)
Day 2 started off with a science fiction dream becoming reality: putting telescopes on the far side of the Moon. Jack Burns, Professor Emeritus at University of Colorado, Boulder, detailed the actual plans to do so in this morning’s plenary. This isn’t a new idea — radio telescopes on the lunar far side were envisioned even before the Apollo 11 landing. Science fiction author Arthur C. Clarke (most famous for 2001: A Space Odyssey) at the time said, “In a few generations, all serious astronomy will be with telescopes on the Moon or in space.” Burns noted that “it has now been a few generations” and we have incredible space telescopes like JWST, leaving only the Moon part left to do.
What makes the lunar far side so appealing is that it’s uniquely radio quiet, with a dry and stable environment and no ionosphere to generate interference. There’s one particular signal radio astronomers and cosmologists want to chase: the well-known 21-centimeter line, which can act as a cosmological probe to explore the dark ages and reionization in the early days of the universe.
NASA is already going back to the Moon with its Artemis program, and its Commercial Lunar Payload Services initiative partners with private companies to deliver more scientific payloads to the Moon. One such project is Radio Wave Observations at the Lunar Surface of the Electron Sheath (ROLSES), which will fly aboard Intuitive Machines 1 in February. The experiment consists of telescoping antennas which will pop out in a spring-loaded system with a classic spectrometer, and will cover a wide frequency range to detect radio signals from a variety of astrophysical signals. It plans to land at the lunar south pole near Shackleton crater, setting the record for closest landing to the lunar south pole; the Indian Chandrayaan-3 probe recently landed 30 degrees away, and this project will only be 10 degrees away.
ROLSES has many science goals, including measuring the electron sheath created by interactions between solar wind and lunar regolith, which is expected to exist but hasn’t yet been measured. It’ll be observing the galactic radio spectrum, measuring the dielectric constant of the lunar subsurface, and — perhaps most importantly — proving that detecting radio signals from the lunar far side is possible, paving the way for future missions. Although ROLSES’ galactic observations won’t reach the pot of gold at the end of the rainbow (the 21-cm line background measurement), it will help characterize the radio emission of the galaxy, which needs to be known, so the Milky Way can be effectively removed from the foreground of future cosmological observations.
Looking further ahead, the LuSEE-Night mission will work on actually measuring that 21-cm line for cosmology purposes in 2025. In the even more distant future, the proposed FARSIDE project would set up an interferometric radio array on the Moon, and the ambitious Far View project would expand such an array to 100,000 dipole antennas, constructed from resources gathered in situ on the Moon.
Press Conference: Exoplanets and Brown Dwarfs (by Pratik Gandhi)
This press conference had four exciting presentations on brown dwarfs and exoplanets, collectively dubbed “other worlds,” by researchers from the American Museum of Natural History and University of California, Los Angeles!
Extrasolar Worlds Exhibit Exotic Sandy Clouds at the Equator
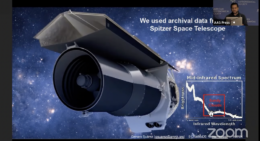
An example BD infrared spectrum from their sample is shown in the bottom right of this photo. The characteristic feature highlighted in red indicates the presence of sandy clouds in the BD atmosphere.
Kicking off the session was Genaro Suarez, a postdoc at the American Museum of Natural History (AMNH), and member of the Brown Dwarfs in New York City group (or BDNYC), who told us about how the equators of exoplanets are often cloudier than the poles! Brown dwarfs (BDs) are considered the link between the lowest-mass stars and gas giant planets — they have insufficient mass to carry out fusion and radiate like stars, but they often have a little too much mass to be considered “planets.” Suarez’s focus is on L-type BDs, which are the hottest category. Using archival data from the Spitzer Space Telescope (the precursor to JWST), they identified a feature in the spectra of BD atmospheres that is indicative of clouds that are composed mostly of sandy grains (see Figure 1)! Additionally, their team found a correlation between the inclination or viewing angle, cloud cover, and color of the BD atmosphere — all of which suggest that BDs have more sandy clouds along their equators than at their poles.
Using Citizen Science to Identify New Ultracool Benchmark Systems
The second talk of this press conference was by Austin Rothermich, a graduate student at the CUNY Graduate Center, and also a member of the BDNYC collaboration. Rothermich’s presentation demonstrated the power of citizen science projects, and how they can lead to important scientific progress with the invaluable contributions of the citizen scientist volunteers! The problem that Rothermich’s research was trying to address is that BDs often have huge degeneracies in their mass, temperature, and age, making it hard to tell these properties apart. Since it’s easier to determine these properties for regular stars (so-called “main sequence stars”), if one finds a regular star near a BD, one can assume they have similar fundamental properties since they must have formed together — then, by measuring the properties of the star, we can infer those of the hard-to-measure BD. This method is therefore called using “ultracool benchmarks”!
Via the citizen science project “Backyard Worlds,” Rothermich’s team was able to incorporate the work of over 175,000 volunteers who analyzed more than 1 million images from the WISE telescope — by flagging the images, the citizen scientists could indicate whether they found a candidate ultracool benchmark system. Their team then followed up 32 of these systems with spectroscopy, and those observations were often aided by citizen scientists.
Rothermich ended the presentation on a poignant and uplifting note, thanking the volunteers who participated for their time and effort, and talking about how his scientific career as an undergrad was also kick-started via the Backyard Worlds project, from the citizen volunteer side!
JWST Indicates Auroral Signature in an Extremely Cold Brown Dwarf
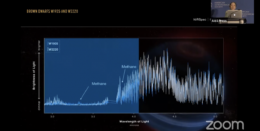
Comparison of two BD spectra showing a number of absorption features due to different molecules like water and CO2. A bump in one of the spectra (in blue) to the left indicates the presence of methane emission.
Next up was Jackie Faherty from AMNH, founder of the BDNYC collaboration, and co-founder of the Backyard Worlds project. Faherty’s results focused on Y-type BDs (the coldest kind), whose surface temperatures range from the setting on a home oven to that of a cold day at the North Pole! Her team used JWST spectra to study the atmospheric compositions of the BDs and found that they were chemically analogous to Jupiter with water ice, methane, carbon dioxide, etc. Faherty showed a comparison of two of their BD spectra, whose “spectra are sculpted by intriguing chemistry,” and discussed how one of them shows a characteristic bump from methane emission (see Figure 3, with the bump towards the left). Normally such a cold object shouldn’t be showing any emission spectra, but using the example of Jupiter they hypothesized that the emission could be due to aurorae at the poles of the BD (similar to those on Jupiter), and that these aurorae could be excited by the presence of a nearby active moon (like Io for Jupiter) or some other internal process. Faherty’s team plans on applying for more JWST time to follow up this system and learn more about whether it has a companion or not. Finally, she ended by discussing how the terms “planet” and “brown dwarf” are often ambiguous, which is why she prefers the term “other worlds” to encompass them all.
WASP-69b’s Escaping Atmosphere Is Confined to a Tail at Least 7 Planet Radii
The final presentation was by Dakotah Tyler, a graduate student at UCLA studying the exoplanet WASP-69b and its curious “tail”-like feature. WASP-69b is one of the 5,000+ known exoplanets, and it belongs to a sub-type known as “hot Jupiters,” or Jupiter-mass planets that orbit really close to their host star and are therefore quite hot. Hot exoplanets can often lose atmospheric mass due to the heating and escape of gaseous material, and Tyler’s team used WASP-69b as a test bed to study exoplanet mass loss in real time. They obtained transit data of the planet crossing the stellar disk, and by looking at helium absorption features in the atmosphere, they found that some of the helium was “blueshifted,” or appeared to be moving fast in our direction — indicating that it had been ejected from the planet’s atmosphere.
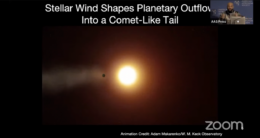
Animation showing what a comet-like exoplanet atmosphere “tail” would look like as it crosses in front of its host star.
The estimated mass loss rate they found is around one Earth mass per billion years, which is higher than previous studies had measured. They also found that although the planetary outflow might have initially been radial or spherical, by coming into contact with the host star’s stellar winds, it got reshaped into a comet-like tail. Finally, Tyler highlighted that their estimate of the size of the tail is roughly 7.5 Earth radii, which is again larger than previously measured for WASP-69b, thanks to the power of JWST!
High Energy Astrophysics Division Bruno Rossi Prize Lecture: Anatoly Spitkovsky (Princeton University) (by Ivey Davis)
In the Bruno Rossi Prize Lecture, Anatoly Spitkovsky of Princeton University succinctly summarized the underlying principle of the field of astrophysical plasmas: “How microscopic processes affect macroscopic astronomical objects.” Few systems (if any) better allow for us to examine the most extreme conditions where plasma physics operates than pulsars. As Spitkovsky recounts, since the discovery of pulsars by Dame Jocelyn Bell Burnell in 1967, the incredible consistency in the arrival time of pulsar pulses have been used for studying the structure of the interstellar medium, served as a test for general relativity, and were used as galactic-scale interferometers for studying gravitational waves as was covered in Monday’s first plenary. While pulsars also serve as a “playground” for theorists due to their extreme magnetic field strengths (billions to trillions of times that of the Sun’s field), densities (100 billion times that of Earth), and rotation rates (as short as a millisecond), there remain questions about the emission processes of pulsars that have persisted since their discovery.
Spitkovsky compares the problem-solving process of pulsar magnetic fields and emission to The Incredible Machine — you keep putting pieces together until something finally clicks and the solution is found, even if a bit convoluted (see associated figure). In the world of pulsar theory, more and more puzzle pieces have been incorporated as the computational power available has increased. For instance, until nearly the year 2000, models of pulsars assumed there was no surrounding plasma; this was relatively computationally inexpensive, but inherently non-representative of pulsar environments. To address this problem, the next piece introduced was a global plasma; this could reproduce how pulsars slow down over time, but could not explain the gamma-ray emission we observe. The most recent — and most computationally expensive — piece has been to transition from a global plasma to individual plasma particles that interact with both the magnetic field and each other. This has served to be the most reliable method to date to reproduce the gamma-ray double-peak light curve and the synchrotron spectrum as observed by the Fermi spacecraft, as well as some other unique pulsar emission.
The conclusion of this work not only seemed to robustly address the age-old problem of pulsar magnetic field morphology and its interaction with plasma, but also fundamentally shifted how we understand where the emission is coming from. We now understand that gamma rays are likely generated by the merger of pockets of plasma, and that this occurs much farther from the surface than we originally assumed the emission to be originating from. This solution was decades in the making, and now that we have it, Spitkovsky is enthusiastic about the new opportunities it affords in addressing other phenomena that are unique to pulsars but inform our overall understanding of plasma astrophysics.
Press Conference: High-Energy Phenomena and Their Origins (by Yoni Brande)
Revealing Dual Quasars and Their Host Galaxy With JWST and ALMA: Yuzo Ishikawa (Johns Hopkins University)
Quasars, ultra-bright actively accreting supermassive black holes (SMBHs), are some of the brightest objects in the sky, often totally outshining the rest of the light from their host galaxies. Most galaxies have a single SMBH in their centers, but some galaxies have two! Yuzu Ishikawa and his collaborators presented their studies of “dual quasars.”
As the name implies, dual quasars have two accreting SMBHs, either as part of two merging galaxies or both in a single galaxy. Most of these have been observed at low redshift, but the dual quasar population at redshifts greater than 1 is not well understood. Perhaps there’s a connection between galaxy mergers and quasar triggering, or the properties of their host galaxies.
To answer these questions, Ishikawa and collaborators studied one particular dual quasar, DQ J0749, at a redshift of 2.17 (or over 10 billion years ago!). The quasars are separated by 3.5 kpc, and the system was discovered with the Hubble Space Telescope. Ishikawa was awarded JWST time to observe the system and found that extended H-alpha emission implies there’s a single host galaxy (a rotating disk!) and that the host is forming lots and lots of stars! The quasars in the system are pretty similar, possibly both accreting gas from the same region in the host. These observations are some of the best-quality data available on these enigmatic high-redshift systems, and Ishikawa and his collaborators hope to learn more about these with future observations.
Breaking Cosmic Scales: JWST’s Discovery of Unexpectedly Massive Black Holes: Fabio Pacucci (Center for Astrophysics | Harvard & Smithsonian)
There’s a well-known relationship between the mass of a SMBH and the mass of the stars in its host galaxy, with the stars outweighing the SMBH by a ratio of about 1000 to 1. However, there’s a population of “overmassive” SMBHS, which JWST has been finding at high redshift (z > 4). Fabio Pacucci and his collaborators studied these to find out if this is an observational bias, or if these mass ratios are being accurately estimated.
At these high redshifts, JWST is able (to paraphrase Dante) “to see the stars again,” allowing Pacucci and collaborators to estimate the stellar mass ratios in these galaxies. They found that, compared to local galaxies, there is a statistically significant difference in these mass ratios, going from 1000 to 1 nearby down to 100 or even 10 to 1 at high redshift.
These results are suggestive of the history of these black holes: by comparing black holes that started their lives light and those that are seeded heavy, the JWST results imply that these overmassive black holes must have started with heavy seeds.
Revealing the Environment of the Most Distant Fast Radio Burst with the Hubble Space Telescope: Alexa Gordon (Northwestern University)
Astronomical transients are non-periodic events that occur and disappear on short timescales. Fast radio bursts (FRBs) are a relatively new class of transients with enigmatic causes that manifest as highly energetic radio pulses on the scale of milliseconds. Alexa Gordon and her collaborators presented a study of FRB 20220610A, the farthest (z = 1) and brightest (4x brighter than any other) FRB yet observed.
Only about 50 or so FRBs have been localized to specific source galaxies, which is critical to understanding the processes that may produce them. Ground-based followup of FRB 20220610A showed inconclusive results as to its source, only showing either a large amorphous galaxy or a merging cluster of three smaller galaxies. Gordon’s team used the Hubble Space Telescope to re-observe the FRB source, and found that it actually originated from a compact group of seven galaxies that together are about the size of the Milky Way! Less than 1% of galaxies at this redshift occur in groups like this!
These galaxies appear to be tidally interacting, which can trigger star formation. Gordon speculates that this tells us about the source of the FRB, as one potential mechanism for FRBs are magnetars, highly magnetized young neutron stars which have very strong emission and are produced as a result of core-collapse supernovae. Gordon and her collaborators are hoping to continue to observe more of these types of FRBs, especially as upgrades to new observatories come online and continue to discover these sources at even higher redshifts.
Burst Chaser: Unveiling the Mysterious Origin of Gamma-ray Bursts with Citizen Science: Amy Lien (University of Tampa)
Gamma ray bursts (GRBs) are the most powerful explosions in the universe. A typical GRB outshines every other source in the gamma-ray sky, combined! The Swift Observatory, NASA’s premier gamma-ray observatory, has detected more than 1,600 of these events across the sky.
GRBs come in many shapes and sizes, with differing energies and pulse traces, with short GRBs (< 2 seconds) typically thought to be produced by mergers of compact objects (like neutron stars or black holes) while long GRBs (> 2 seconds) are thought to be produced by supernovae. However, these are not hard classifications, and Lien and her colleagues turned to citizen science to more accurately classify these energetic signals to more accurately characterize them by their physical origins.
A test program showed that volunteer human classifiers (made up of a beta test group of astronomers and the interested public) perform better than existing GRB classification codes for sorting these traces by their observed shapes. Now, Lien and her collaborators are taking their program to the public, using the Zooniverse website to host their GRB classification data. You can join this project at https://www.zooniverse.org/projects/amylien/burst-chaser.
Seeing Our Sun Through a New Lens: The First Large Catalog of Hot Thermal Solar Flares: Aravind Valluvan (University of California, San Diego)
Solar flares, random broadband energetic bursts from the Sun, are a critical component of space weather. These events typically last about 20 minutes or so, but they could be as short as a few minutes or as long as half a day. These are the most energetic explosions in the solar system, and they have long been known to pose a threat to electronic communications infrastructure and sensitive operations like high-altitude aviation. These effects are important to study, in order to be able to predict them in advance and mitigate their effects.
Aravind Valluvan, from UCSD, studies these flares, looking specifically at “hot thermal flares”: flares with relatively symmetric light curves. The typical “impulsive” flare has a very quick, energetic rising phase and a much more gradual decay, while hot thermal flares increase and decrease in intensity on roughly the same timescales.
Valluvan says that while typical impulsive flares are caused by rapid magnetic field reconnection quickly dumping energy into the solar corona, hot thermal flares instead may be a result of slower magnetic reconnection processes, or slower energy transfer to the corona. Valluvan’s group observed 2,200 of these hot thermal flares and their catalog will be critical to properly interpreting these flares’ formation mechanisms. Are there connections between hot thermal flares and other solar phenomena like coronal mass ejections or solar particle events? Maybe! ISRO’s recently launched Aditya-L1 mission recently reached its final orbit, where it will use its X-ray spectrometer and ultraviolet imager to study the Sun and its energetic outbursts, just in time for solar maximum. Valluvan is hopeful that this mission will help us finally answer some of these critical questions.
Annie Jump Cannon Prize Lecture: Theories of Planet Formation, Eve Lee (McGill University) (by Briley Lewis)
For the 2022 Annie Jump Cannon Prize lecture, Eve Lee discussed the detailed (and quite complicated!) theoretical physics of planet formation. The planet populations we observe, she reminded the audience, are the consequences of coagulation and chemistry in the protoplanetary disk, disk–planet interactions, orbital dynamics, and atmospheric processes. She considered all these factors to try to explain: (1) a set of three puzzling (and seemingly contradictory) observations, (2) the origin of the Neptune desert, and (3) the favored location of Jupiter-like planets.
Let’s start with the trio of puzzling observations. First, outer giant planets appear to be more common around higher-mass stars. Second, inner super-Earths are more common around lower-mass stars. Third, systems with outer giants tend to have inner super-Earths. At first glance, it seems like the third observation is in tension with the first two — but Lee described in her talk (and also in a paper published last year) why these observations are actually reconcilable, and explainable by physics. Outer giants have inner super-Earths, because if a disk is large enough to form a giant planet, it also likely has enough mass to form a smaller super-Earth. Large stars have larger disks, so they form big planets, and small stars are able to convert pebbles to planetesimals more efficiently because they are cooler.
The Neptune desert — a notable lack of planets similar in size to Neptune in a region close to the host star — isn’t the result of just one factor, according to Lee. Part of the gap is from planets that just formed like that, lacking in metals to effectively cool themselves and accrete a gaseous envelope. Then, the gap is further carved by atmospheric evaporation caused by the star.
Finally, to determine why Jupiters so often form between 1 and 10 au, she considered what’s going on physically at each bound of that range. At the inner boundary, migration cuts off the ability for large planets to exist there. At the outer boundary, dust grains drift faster than they can grow, limiting the size of planets that can form.
Lee’s talk was a great reminder that planetary systems are extremely complex, and we have so much left to learn about how they become the worlds we observe — and even about how our own planet came to be.
Plenary Lecture: Sukanya Chakrabarti (University of Alabama, Huntsville) (by William Lamb)
Our galaxy rotates faster than it should if it only contains observable matter. This is why astrophysicists predict the existence of a dark matter halo surrounding the Milky Way to account for the extra, unobserved mass that must be there. The problem is, we don’t know much about dark matter because we have never observed it directly, and we don’t know how it is distributed in our galaxy. Sukanya Chakrabarti’s talk focused on probing dark matter by directly measuring the acceleration of stars within the Milky Way’s gravitational potential, which should bring further enlightenment on the effects of dark matter within a galaxy.
Traditionally, astrophysicists would estimate the acceleration induced on galactic stars by gravity by taking observations of the velocities and positions of those stars. This requires the assumption that galaxies are in equilibrium, where the gravitational potential and hydrostatic pressures that affect the motion of stars within the galaxy are balanced. However, galaxies are dynamic — interactions with satellite dwarf galaxies perturb galaxies over time. By directly measuring the acceleration of stars, Chakrabarti’s research enables the study of “real-time galaxy dynamics.”
Her group achieves this in four ways. First, they run dynamic simulations of the Milky Way, as opposed to simulations featuring static potentials. Second, they take high precision radial velocity observations, requiring a precision of 10 cm/s on velocity measurements, to measure the acceleration of some galactic objects. Third, they use pulsar timing measurements, such as those from NANOGrav, to effectively create a “galactic accelerometer” to measure the accelerations of pulsars. And fourth, they take measurements of the mid-points of exoplanetary eclipses, requiring a precision of 0.1 second across a 10-year baseline, to measure the accelerations of stars with exoplanets.
These measurements are extreme and on the edge of the technical capabilities of modern instruments and data analysis techniques. However, Chakrabarti is showing that this is possible, and hopefully insights from her work will further our understanding of dark matter in our galaxy.