Editor’s Note: This week we’re at the virtual 237th AAS Meeting. Along with a team of authors from Astrobites, we will be writing updates on selected events at the meeting and posting each day. Follow along here or at astrobites.com. The usual posting schedule for AAS Nova will resume on January 19th.
Henry Norris Russell Lectureship: Comets, Unseen Planets, and the Outer Fringes of the Solar System (by Haley Wahl)
The first plenary of the third day of AAS 237 was given by Scott Tremaine of the Institute for Advanced Study, who gave the Henry Norris Russell Lecture, an honor awarded to a scientist on the basis of lifetime eminence in astronomical research. His talk focused on one of his many interests: comets. Dr. Tremaine started off by giving a brief overview of comets, which are chunks of rock and ice with a radius of a few kilometers. They are thought of as “rubble piles” because of their low density and are believed to be leftover small bodies from the formation of our solar system. Atmospheres (coma) and tails appear when a comet approaches the Sun and once a comet becomes visible, it can survive for more than 100 orbits.
Dr. Tremaine then dove into discussing the energy of comets and how we can predict where comets will be and where they come from by looking at how much energy they have when passing through our solar system. He then spoke about the Oort Cloud, a region far beyond the orbit of Pluto where a lot of comets are thought to originate. The Oort Cloud is far enough from the Sun that kicks from passing stars and torques from galactic tides can mess with the orbits of the comets in the Cloud, meaning that comets can be removed from it. There is a standard model of the formation of the Oort Cloud, which contains assumptions and initial conditions for how comets form. By using that theory and doing simulations, astronomers can pretty well determine the properties of the inner Oort Cloud. Like the standard model for particle physics, the standard model for the Oort Cloud isn’t perfect.
There are parallels between the standard model of particle physics and the standard model of comets! pic.twitter.com/xSlZanYDDH
— astrobites (@astrobites) January 13, 2021
The final part of Dr. Tremaine’s talk focused on the possibility of another major solar system body existing, an idea motivated by comets on very large orbits — for instance, Sedna!
Sedna and others like it do not follow the standard model … so how did they end up on their current orbits? The standard model is based on the planets and many test particles, so maybe it is oversimplified and we should factor in things like kicks from passing stars and the galactic tide to explain comet orbits. Simulations show that there is a possible undiscovered planet that is ~0.1–1 solar mass that is within 250 au of the Sun, nicknamed “Planet X” (this concept is also sometimes referred to as “Planet Nine”). There is a lot of speculation about whether or not Planet X exists and, if it does exist, where it came from.
There has been a lot of speculation about Planet X, here is a little bit more information! pic.twitter.com/hE3WSCoJU4
— astrobites (@astrobites) January 13, 2021
Dr. Tremaine ended his talk by summarizing that the standard model of the formation of the Oort Cloud explains most but not all properties of comets and that there are possible hidden planets out there. In the next decade, we could improve constraints on Planet X or discover it, but we will not be able to rule it out. He finished by leaving up an XKCD comic:
Interview of Scott Tremaine and session live-tweeting by Haley WahlPress Conference: Bursting Magnetars (by Ellis Avallone)
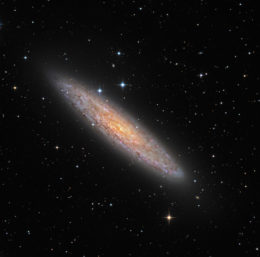
NGC 253 is a bright spiral galaxy located about 11.4 million light-years away in the constellation Sculptor. [Dietmar Hager and Eric Benson]
So-called giant magnetar flares occur when starquakes cause a magnetar’s strong magnetic field to rearrange. The Sculptor galaxy magnetar underwent such an event, producing a short burst of gamma rays that was detected by five of the satellites in the Interplanetary Network (IPN) — an array of telescopes essential for localizing gamma-ray bursts. It has long been suspected that some short gamma-ray bursts originate from magnetars, and the detection of one in a nearby galaxy confirmed this suspicion. Although giant magnetar flares represent the most extreme release of magnetic energy we observe in magnetars, we can only observe them out to about 50 million light-years. The triangulation of the signal with the IPN showed that the burst had to have come from the Sculptor galaxy. When triangulating a signal, there is always a chance of a false association, where a background signal is incorrectly associated with an object. However, the chance of this happening for this magnetar flare is about 1 in 230,000, which further confirmed the location of the flare.
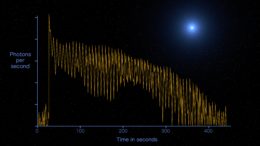
The distinct light curve of a giant flare from a magnetar includes a sudden peak followed by a long, spiky decay tail. The spikes are caused by the gradually cooling hot spot on the magnetar’s surface rotating in and out of view. [NASA’s Goddard SFC]
What does this event mean in the context of other magnetar flares? Dr. Eric Burns from Louisiana State University wanted to find out if giant magnetar flares were being buried in short gamma-ray burst signals, which more frequently originate from neutron star mergers. Although the sample of giant magnetar flares only consists of a handful of events, it is large enough to provide insight into the occurrence rates of these events. With this information, Burns and his team determined that giant magnetar flares occur more frequently than supernovae, at least in the local universe.
Finally, Dr. Victoria Kaspi from McGill University commented on this result and what it means for other areas of astronomy. Most notably, giant magnetar flares are a potential source of fast radio bursts (FRBs), whose origin is still unknown. The occurrence rate for giant magnetar flares found by Burns is consistent with FRB occurrence rates. However, more work needs to be done to confirm that FRBs originate from magnetars.
Press release for the briefing
Live-tweeting of the briefing by Ellis Avallone
STScI Town Hall (by Mike Foley)
The Space Telescope Science Institute (STScI) manages many of the space telescopes, including the Hubble Space Telescope (which has been operational since the 1990s), James Webb Space Telescope (JWST), and Nancy Grace Roman Space Telescope (the latter two of which have yet to be launched). STScI also maintains the Barbara A. Mikulski Archive for Space Telescopes (MAST), which distributes data from over 20 astronomical missions. The Town Hall today focused on a Hubble program, planning for the Roman Telescope, and recent results from an advanced optics lab.
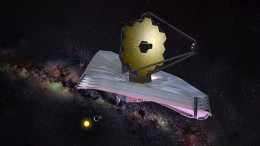
An artist’s illustration of the James Webb Space Telescope, a joint effort between NASA, the European Space Agency, and the Canadian Space Agency. [NASA/JWST]
He was followed up by Julia Roman-Duval, who presented updates about the ULLYSES Hubble program. ULLYSES (the Ultraviolet Legacy Library of Young Stars Essential Standards), a proposed mission that will last three years, represents the largest single Hubble program (observing run) ever, and its span will include nearly 1,000 Earth-orbits of Hubble, providing observations that can be used to study both high- and low-mass young stars. Around 500 of these orbits will be used to extend the spectroscopic library of O and B stars to low metallicity. These data will give important information about the properties of these massive stars, spectral templates for certain stellar populations, chemical information, and characterization of some galaxy-scale outflows in the circumgalactic medium. The other 500 orbits will be used to study T Tauri stars in eight nearby star-forming regions, allowing for an unprecedented look into the lives of young, low-mass stars.
Karoline Gilbert spoke next about the Roman telescope and how the community can begin to engage with it. Roman will launch in the mid-2020s and have a huge field of view, nearly 100 times larger than Hubble’s (which is currently about 1/10th the diameter of the full Moon)! All data from the Roman space telescope will be publicly available with no proprietary period, meaning anyone can access and use the data as soon as it is downloaded. Roman will be able to perform tasks much quicker than previous surveys; for example, it will image galaxy fields over 1,000 times faster than the CANDELS survey! Beth Willman, the Chair of the Roman Space Telescope Advisory Committee, and Megan Donahue, the Chair of the Roman Science Interest Group, are two points of contact for the community and anyone interested in getting involved with Roman.Rémi Soummer finished up the Town Hall with updates from the Russel B. Makidon Optics Laboratory, a state-of-the-art optics lab at STScI created in 2013 to advance technologies for future generations of space telescopes. Recently, they worked on a project aimed at direct imaging of exoplanets, which is extremely difficult and requires sensitive technology. Some of their recent projects are aimed at enabling direct images of exoplanets. Using their equipment, PhD student Susan Redmond recently showed that they could maintain excellent contrast in coronagraph images, even when perturbations are introduced into the system over time. The lab has made a number of student-led improvements that will be relevant to future missions, and they are now operating remotely and have developed ways to maintain experiments throughout the pandemic.
Live-tweeting of the session by Mike Foley
Plenary: Distinguishing Black Hole Binary Formation Channels With Eccentricity Measurements and Other New Gravity Wave Probes (by Luna Zagorac)
The second plenary of the day, re-named “What (Else) Can We Learn About Binary Black Holes?,” was given by Professor Lisa Randall of Harvard University. She noted that the work she was presenting was done with Professor Zhong-Zhi Xianyu of Tsinghua University, as well as with students Alexandra Shelest and Nicholas Deporzio. Dr. Randall started off by introducing a particle physicist’s approach to a particular astronomical data set: LIGO’s observations of stellar-mass black hole mergers via gravitational waves! Data sets in particle physics are scarce, Randall argued, so we eke out the most physics possible from them — and we should do the same with LIGO’s data. With this in mind, what new information can we learn from LIGO data? Randall refers to this approach as BSA: Beyond Standard Astronomy.
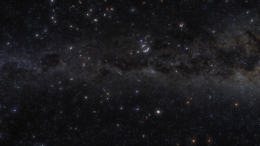
The dense and chaotic cores of globular clusters, as shown in this simulation still, may provide an ideal environment for black holes to interact dynamically and merge. [Carl Rodriguez/Northwestern Visualization]
Thankfully, there is a mechanism that produces extremely eccentric orbits, called Kozai–Lidov. It is a feature only of the three-body system, making it extremely interesting for probing the dynamic formation channel when used with eccentric gravitational wave events such as GW190521. Furthermore, the launch of the gravitational wave observatory LISA will help the situation in two ways.
First, while we can measure eccentricity statistically with Advanced LIGO (in terms of sheer number of events), LISA will allow us to actually measure the orbital motion directly. Furthermore, because eccentricity can change really quickly while the black holes are inspiraling, we could be able to see the eccentricity variation in the LISA window over several years — a direct observation of the Kozai–Lidov effect!Second, binaries that start with small eccentricities will be imaged with both LIGO and LISA, allowing us a multichannel view of the merger. In contrast, binaries with large eccentricities will only be seen in a single channel, thus allowing us to discriminate between dynamic and other formation channels. Ultimately, this may mean determining the origins of stellar-mass black hole binaries!
Live-tweeting of the session by Luna Zagorac
Press Conference: Dizzy with Data for DESI (by John Weaver)
The second press conference of the day was all about the Dark Energy Spectroscopic Instrument Survey, or DESI for short. The DESI Survey is conducted at the Mayall 4-meter telescope at Kitt Peak National Observatory and has been running since 2019. Its goal is to map the universe to better understand the nature and origin of, well, dark energy. But as we will see from the press conference today, whenever we peer into the night sky we find lots more than we bargained for. What more could we want? The Data Release 9 is out today.
Co-Project Scientist David Schlegel (Lawrence Berkeley National Laboratory) started us off with a brief overview of the survey. The DESI survey works by first collecting an incredible amount of images of the sky. A team of 150 astronomers help to condense 1,400 nights on 3 telescopes totalling a whopping 1 petabyte of data into vast maps of the night sky. They are aided by immense computing power with over 100 million supercomputer hours spent just on DESI. You can view the polished images here. Although the survey is not yet complete, there are already many exciting discoveries being made. Press release
Lots of fascinating "discoveries waiting to happen" in these images. These will be the focus of another talk in the conference! Have a look at this "galaxy-sized telescope" which helps us peer into the more distant universe. #AAS237 pic.twitter.com/VDbRuZ3Hhn
— astrobites (@astrobites) January 13, 2021
Strong gravitational lenses help magnify distant background galaxies using the power of gravity. Xiaosheng Huang (University of San Francisco) explains that thanks to the DESI area, they are able to find many more of these incredibly rare lenses, affording new opportunities to explore the very distant universe. These “galaxy-sized telescopes” are bulked up by their massive dark matter halo which helps bend light from more distant background galaxies. Since there may be many images of the background galaxy caused by light traveling along different paths around the lensing galaxy, one unique application is to study supernovae by measuring the time delay between the supernovae seen in the duplicate images. This can ultimately help us to understand the nature of cosmic expansion. Press release
They now have the BEST EVER 3D map of brown dwarfs in the solar neighborhood. Incredible, with lots of discoveries to come. #AAS237 pic.twitter.com/bl1PFJZ8TP
— astrobites (@astrobites) January 13, 2021
Cold brown dwarfs are some of the most bizarre and yet fascinating sub-stellar objects in the universe. Aaron Meisner (NSF’s NOIRLab) has collaborated with a huge team of citizen scientists through the Backyard Worlds: Planet 9 project, launched in 2017 at Zooniverse, and has since collected more than 7 million user ‘classifications’ from 150,000 contributors. The result? They have constructed the most complete 3D map of brown dwarfs in the solar neighborhood, selected from images from NASA’s WISE satellite together with the data from DESI. Predictions suggest that our solar neighborhood should host a variety of stellar types, brown dwarfs included. However, the results from Backyard Worlds suggest otherwise: they find the nearest brown dwarf seriously far away at 7 parsecs distance. That may mean that our solar neighborhood is tantalizingly devoid of brown dwarfs, or they’re possibly just too faint to be detected. The citizen scientists not only get to share in the limelight of the discovery, but they have the opportunity to work on projects connecting them to some of the premier facilities in all of astronomy. Press release
This is only a very small portion of the total @desisurvey data footprint. So many galaxies! DESI helps by adding even more spectroscopic redshifts to help pin down their distances. #AAS237 pic.twitter.com/SKP0C2OZ21
— astrobites (@astrobites) January 13, 2021
Black holes in galaxies also seem to be missing. The X-ray emission from all of the monster black holes found so far doesn’t add up to what we see from the cosmic X-ray background. Stephanie Juneau (NSF’s NOIRLab) has worked to correct this dire picture. By using the incredibly wide imaging from the DESI survey, she and her team have tracked down some of these missing black holes. The trick? Combining multiple imaging surveys with spectroscopic follow-up. This way they can identify candidate black holes from the images and then use DESI spectroscopy to confirm them. But the X-ray emission from black holes likes to ‘flicker’ on and off, making them even more difficult to find. If the survey can confirm all of their candidates, Juneau and her team can better understand how often black holes flicker, which has the potential to impact how we understand the growth of black holes and their connection to their host galaxies.
Live-tweeting of the briefing by John Weaver
Annie Jump Cannon Award in Astronomy: Hazy Views of Nearby Worlds: Standing Between Two Eras of Exoplanet Characterization (by Michael Hammer)
The last plenary talk of the day was given by Professor Caroline Morley (Univ. of Texas at Austin), this year’s winner of the Annie Jump Cannon Award in Astronomy. An expert on characterizing exoplanet atmospheres, Morley “has advanced our understanding of clouds and photochemical hazes” and “paved the way for the robust detection of water and other molecules in exoplanet atmospheres.” Above all, she is also an Astrobites alum who helped us cover these very AAS meetings as recently as five years ago! Over the past decade, Morley has modelled a wide variety of atmospheres of (1) different-sized planets, and (2) different-temperature brown dwarfs. After years of relying mainly on the Kepler and Hubble space telescopes for her research, she is now looking forward to the new era of TESS and JWST! In the coming years, TESS will hunt down virtually all of the planets closest to Earth that are best-suited for having their atmospheres characterized, while JWST will enhance our capabilities for probing molecular features and other facets of these atmospheres like clouds and hazes with its much larger 6.5-meter aperture and a wider range of wavelengths into the mid-infrared.The three-part overarching goal driving the field of exoplanet atmospheres is to find molecular biosignatures in the atmosphere of an Earth-like planet around a solar-type star. Before we can do that though, we had to start out by making concessions on all three parts. Most studies of exoplanet atmospheres are based on transmission spectra (see Morley’s own bite for an overview, and here and here for more recent updates) that are taken as a planet transits in front of its star. Similar to the limitations of detecting planets through transits, planets that produce deeper transits yield better-resolved spectra, making it easier to detect features in atmospheres of planets bigger than Earth or around stars smaller than the Sun. Meanwhile, planets that have thicker atmospheres (>1% by mass) than Earth (0.0001% by mass) are also easier to resolve. Beyond just biosignatures, we want to characterize what planetary atmospheres are made of in general.
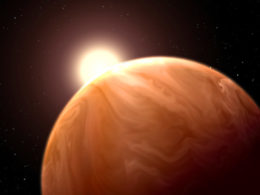
Artist’s impression of a cloudy exoplanet. [NASA/ESA/G. Bacon, STScI/L. Kreidberg and J. Bean, University of Chicago/H. Knutson, Caltech]
Beyond planets, another focus of Morley’s research has been brown dwarfs. The atmospheres of free-floating brown dwarfs are easier to resolve than those of planets around stars because their spectra are not contaminated by nearby starlight. And, more importantly, they may shed some insight on what planetary atmospheres are like because the coldest ones that we only started discovering this past decade (Y-type) have similar temperatures to planets — at least one is even colder than the Earth! Morley and her collaborators have shown that the atmospheres of Y dwarfs can have thick methane hazes and be out of equilibrium.
Sounds exciting? The AAS certainly thinks so, in that they decided to give next year’s Annie Jump Cannon Award to one of Morley’s collaborators: Laura Kreidberg. Stay tuned for more developments in the field of exoplanet atmospheres — TESS and JWST will certainly provide them!
Interview of Caroline Morley by Briley Lewis
Live-tweeting of the session by Michael Hammer
Hearing the Light: How Sonification Makes Astronomy More Accessible (by Abby Waggoner)
Clara Braseur (Space Telescope Science Institute) & Jenn Kotler (Space Telescope Science Institute) hosted this afternoon’s session on sonification.
As beautiful as the images, plots, and figures we use for astronomy are, visual based astronomy presents a barrier for individuals who have visual impairments or are blind. Sonification is a process that converts visual images into sounds, thus making astronomy more accessible. For a (really awesome) example, check out this NASA website for some examples that bring three astronomical objects to our ears.
Not only does sonification make astronomy more accessible, but it also presents a new method of interpreting data. Our eyes are able to process roughly 100 frames per second, whereas our ears are able to process over one million frames per second, thus detecting information that our eyes may have missed. We are also able to filter out background sounds and noise more easily in audio rather than visual form a phenomenon known as the cocktail effect — which can make finding patterns in noisy data much easier.
Sonification is a really cool method, but how does one go about doing it? Thanks to the program Astronify, sonification of your own data is super easy to do. Astronify is a python based code and can be installed on any computer for free using a simple installation command. You simply give it a table of data, such as flux and time, and let it run! Astronify is currently ideal for sonifying lightcurves, spectra, or any other 2D plot, but the Astronify team is working on introducing more dimensions and error bars on the sounds. Check out the two videos below for the sonification of lightcurve with no flare and a lightcurve with seven flares using Astronify.
Sonification is an exciting tool we can use to make astronomy more accessible, and thanks to Astronify, it is becoming a process we can apply to our own data.
1 Comment
Pingback: Protokoll der 237. – virtuellen – Tagung der AAS | Skyweek Zwei Punkt Null