Simplifying a problem to make it solvable is a classic trademark of scientific modeling. But what happens when cows simply aren’t spheres?
The Perpetual Struggle: Accuracy vs. Feasibility
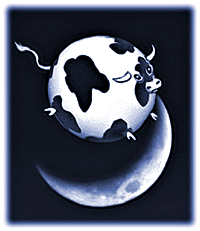
Theoretical models can fall into the trap of becoming spherical cows — they assume simplifications that render them no longer realistic. [Ingrid Kallick]
A major challenge for theorists is to develop models that are as realistic as possible, but are still simple enough to be solvable. This often requires making simplifying assumptions, turning complex systems into spherical cows. At times, these assumptions might be good approximations. At other times, they might be oversimplifications that cause us to misinterpret observations.
In a recent study, scientist Jack Scudder (University of Iowa) challenges an especially long-standing assumption used in many models of astrophysical and space plasmas.
Equilibrium or No?
Astrophysical and space plasmas are soups of ionized gas found throughout the universe, from supernova remnants to the intergalactic medium, from the Sun’s atmosphere to Earth’s magnetosphere. We can observe the photons emitted by these plasmas, and with the help of theoretical models, we can use these observations to remotely infer the properties of the astrophysical kitchens in which they were made.
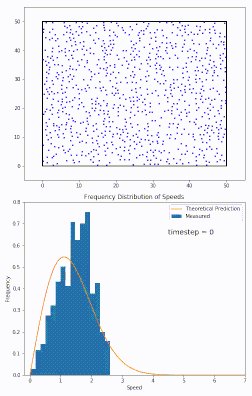
Simulation showing a 2D gas relaxing toward a Maxwellian distribution as its particles collide. [Dswartz4]
Is the LTE approximation reasonable to assume for astrophysical and space plasmas? Scudder argues that it often isn’t, especially in astrophysics where gravity requires strong spatial gradients in plasma properties that aren’t permitted in thermodynamic equilibrium. This means we’re likely interpreting observations incorrectly.
A New Solution
So if LTE isn’t a good approximation, what should we be using instead? Scudder has developed a model he calls SERM — Steady Electron Runaway Model — that generalizes the Maxwellian distribution for astrophysics, but reduces to it when spatial gradients are presumed absent. This model, he says, can be used to interpret observations of plasmas that are not necessarily in LTE.
To test his model, Scudder applies it to experimental measurements of the solar wind — the plasma streaming off of the Sun’s surface and suffusing interplanetary space. He shows that SERM predictions neatly match observations, and the model additionally explains a number of odd solar wind features that have puzzled scientists for decades.
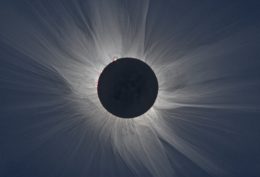
A view of the solar corona during the 2015 total solar eclipse in Svalbard, Norway. [S. Habbal, M. Druckmüller and P. Aniol]
There’s more work to do to flesh out this model, and it is admittedly more complicated to work with than models that rely on the simplifying assumption of LTE. But Scudder’s work shows that SERM may be the next step needed in theoretical modeling to move the field forward.
Citation
“Steady Electron Runaway Model SERM: Astrophysical Alternative for the Maxwellian Assumption,” J. D. Scudder 2019 ApJ 885 138. doi:10.3847/1538-4357/ab4882