The 234th meeting of the American Astronomical Society will take place June 9–13 in St. Louis, MO. In advance of the meeting, Astrobites authors have conducted interviews with some of the meeting’s keynote speakers to learn more about their research and careers. We’ll be publishing those interviews here over the coming days as part of our #AAS234 series!
Professor Yacine Ali-Haïmoud (interviewed by Kate Storey-Fisher)
Primordial black holes are like dark matter particles; spinning grains of dust are like binary black holes; the gravitational wave background from pulsar arrays is like the cosmic microwave background from radio interferometry. This is how Yacine Ali-Haïmoud, Assistant Professor of Physics at New York University, sees the world. “I like to look for analogies between things which seemingly have nothing to do with one another,” he says. Physics is full of phenomena in far-flung regimes that follow similar laws. Ali-Haïmoud, who is giving the Warner Prize Lecture at AAS 234 this month, follows these physical analogs to theorize about cosmological imprints on observables.
A primary interest for Ali-Haïmoud is primordial black holes (PBHs), which have recently made a comeback as a dark matter candidate. This idea was first floated in the 1960s, and became popular again after the 2015 detection of black holes by LIGO (see this astrobite). These hypothetical black holes may have formed via gravitational collapse in the early universe, and could range from the mass of an asteroid to thousands of times the mass of the Sun.
Ali-Haïmoud is using the cosmic microwave background (CMB) to place constraints on PBHs. Black holes accrete nearby gas, heating up the gas and injecting more energy into the surrounding plasma. This leads to a change in the ionization history of the plasma, which changes the time of last scattering of CMB photons. We would then see a signature of this effect in the statistics of the CMB temperature anisotropies that we measure today. Ali-Haïmoud and others have already constrained PBH parameter space with Planck data, but they have not yet found evidence that PBHs contribute to the dark matter budget. “What I’ve been working on is building more precise translations from microphysical models to observables,” Ali-Haïmoud says, “such that if you do see something that departs from the standard cosmology, you should be able to actually pinpoint more accurately what possible dark matter model could cause this.” Future CMB maps from the Simons Observatory will probe this effect with higher sensitivity and hone in on dark matter candidates including PBHs.
Ali-Haïmoud is also interested in the story of interstellar dust, approaching it both theoretically and observationally. Large dust grains absorb ultraviolet photons and heat up, exciting the internal degrees of freedom of the grains. This is re-radiated in the infrared, but observers have also detected an unexplained bump in microwave emission. One hypothesis is that some dust grains are spinning at just the right frequency to produce this emission. A class of molecules called polycyclic aromatic hydrocarbons (PAHs) might fit the bill; Ali-Haïmoud describes these as “the dark matter of interstellar physicists.” We have a lot of indirect evidence of these large chemical compounds in the interstellar medium, but it is difficult to identify the exact types of molecules and their abundances.As a step towards identifying interstellar PAHs, Ali-Haïmoud worked out the spectrum that PAH dipoles would produce. He notes that the electric dipole moments of the PAHs are analogous to the mass quadrupole moment of a binary black hole. But unlike black holes, PAHs are small enough to be governed by quantum laws, so they undergo discrete rotational transitions. These manifest in spectral lines that clump together in comb-like features, producing unique signatures for each molecule. Ali-Haïmoud went observing to hunt for these features (“I’m pretty proud of this because I’m a theorist,” he says), taking data of a cloud known to host anomalous emission. Alas, he didn’t find any spectral comb signatures, but he used this non-detection to place limits on the amounts of certain PAHs. “One of the things I’m hoping is that other people will do this kind of observation, which no one had done before,” he says.
Along a different vein, recent discussions with colleagues got Ali-Haïmoud interested in pulsar timing arrays (PTAs) as a way to map out the gravitational wave background. PTAs measure frequencies far below LIGO by monitoring pulsars — stable, rotating neutron stars that emit a beam of radiation. This beam appears as a pulse when it swings across the detector, and in the absence of gravitational waves, PTAs would detect pulses at extremely regular time intervals. A stochastic gravitational wave background, such as that from binary supermassive black holes, would perturb these arrival times. Ali-Haïmoud is working with PTA experts and bringing in his own expertise to develop the formalism for mapping the gravitational wave sky with these arrays. He compares this technique to the interferometric mapping of the electromagnetic background (the CMB) with arrays of detectors, which provided the first constraints on anisotropies and later polarization. “Here it’s philosophically similar, what we’re trying to do,” he says, “in the sense that we’re trying to understand what are the geometric properties of the maps that one could obtain from arrays of pulsars.”
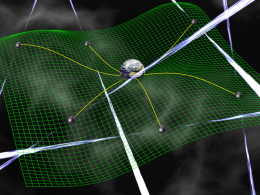
An artist’s illustration showing how a network of pulsars could be used to search for the ripples in space-time. [David Champion/NASA/JPL]
For undergraduates aspiring to a career in academia, Ali-Haïmoud emphasizes the importance of talking to professors and others in your field of interest. “Towards the last couple years of undergrad, I think it’s good to get in touch with faculty members to try and go beyond just what you learn in class, and try to see connections,” he says. More generally, “It’s always better to go and talk to people, whether they’re big shots or not big shots. You always learn more — even if you just have some poorly phrased idea, you always learn more by sharing this [idea]with people and getting some kind of feedback.”
After completing his graduate studies at Caltech, Ali-Haïmoud completed postdocs at the Institute for Advanced Study and Johns Hopkins University. In 2017, he became an Assistant Professor of Physics at New York University. “It’s good to be aware that it’s a long road and it’s not guaranteed for anyone, that there’s unfortunately a huge amount of luck involved,” Ali-Haïmoud advises. “So professors are really people who are hardworking and were also extremely lucky.”
Hear from Ali-Haïmoud at his Helen B. Warner Prize Lecture, “Hunting for Dark Matter in the Early Universe,” on Monday, June 10 at 12:20pm at #AAS234.
Professor Anthony Yeates (interviewed by Mia de los Reyes)
The Sun is the single most important astrophysical object for life on Earth. Its light is the basis for nearly all the food and energy on Earth; its heat provides just the right amount of warmth to keep Earth’s oceans liquid. Even the passage of time has been historically marked by the Sun — the radioactive decay of atoms might be a more fundamental unit of time, but it is the Sun’s motion relative to the Earth that defines the natural timescales of life.
Yet despite centuries of human observations, the Sun still harbors some secrets. “You tend to think that the Sun is the nearest astrophysical object,” says Anthony Yeates, so we should understand it pretty well. “But […] it’s even more complicated than we thought.”
Yeates, an associate professor from Durham University, studies the structure and evolution of the solar magnetic field, one of the most enduring mysteries about the Sun. As this year’s winner of the Karen Harvey Prize, he will give a prize lecture at #AAS234 addressing the question “Where Do Solar Eruptions Come From?” The answer to this question — perhaps unsurprisingly, given Yeates’ area of expertise — has to do with the Sun’s magnetic field.
Unfortunately, the solar magnetic field cannot be directly observed. So, Yeates says, “you need numerical models to try to get a handle on what it actually looks like.” These models aim to piece together a patchwork of indirect observations. For example, we can directly measure the solar magnetic field at the location of Earth, then try to extrapolate it back to the Sun, where we can compare with data about the Sun’s surface obtained from instruments like the Solar Dynamics Observatory.
Patterns from the solar magnetic field can also imprint themselves on the Sun’s corona (lower atmosphere), leading to observable energetic phenomena. One such phenomenon is a so-called coronal mass ejection (CME), which occurs when a huge quantity of solar plasma is released from the Sun. The energetic output from a CME — up to billions of tons of material! — can, when directly aimed at the Earth, produce geomagnetic storms that disrupt navigational systems, GPS, and power grids.
CMEs are thought to result from twists in the solar magnetic field. As the Sun’s magnetic field twists into helical structures, it builds up energy until the energy is somehow forcefully released — like a rubber band deformed to the point of snapping.
However, there are still open questions about how this happens, especially on small scales. How are these twists (“magnetic helicity”) injected into the corona? Where are they stored? How does magnetic helicity become concentrated enough to cause an eruption?
These are the questions that Yeates hopes to answer: “We’re trying to develop mathematical tools for identifying where within the coronal magnetic field you have interesting structure: where the magnetic field is tangled, twisted, where energy is stored.” If we can understand this, Yeates says, we may be able to predict where and when CMEs can occur — and even their internal structure, which may have a significant impact on how strongly they impact the Earth’s magnetic field.Yeates has always enjoyed using mathematics as a tool; as an undergraduate, he studied applied mathematics, originally intending to pursue solid mechanics or fluid mechanics. But when he applied for PhD programs in the UK, he found a research project on the Sun that “seemed interesting,” and he took the opportunity.
“I just drifted into it by accident,” Yeates laughs. “I didn’t know anything about the Sun, I had no life-long ambition to study anything like this. […] I never even studied physics, as such.” He now finds that his mathematical training helps give him another point of view when solving astrophysical problems, though he does think it’s important to understand the background physics.
When asked what advice he’d give to more junior researchers, Yeates says, “Keep better notes! Write down literally everything you do.” And, true to his roots as a mathematician, he adds, “Never believe anything you calculate. Assume you made a mistake … because nine times out of ten, you did make a mistake! No one does things correctly the first time.”
If you’re interested in hearing more about how Prof. Yeates applies mathematics to understand the Sun’s magnetic field, come check out his plenary talk at 4:30PM on Wednesday, June 12 at #AAS234!
1 Comment
Pingback: AAS 234: Welcome!