What happens on the last day of a massive star’s life? In the hours before the star collapses and explodes as a supernova, the rapid evolution of material in its core creates swarms of neutrinos. Observing these neutrinos may help us understand the final stages of a massive star’s life — but they’ve never been detected.
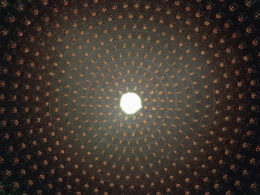
A view of some of the 1,520 phototubes within the MiniBooNE neutrino detector. Observations from this and other detectors are helping to illuminate the nature of the mysterious neutrino. [Fred Ullrich/FNAL]
Silent Signposts of Stellar Evolution
The nuclear fusion that powers stars generates tremendous amounts of energy. Much of this energy is emitted as photons, but a curious and elusive particle — the neutrino — carries away most of the energy in the late stages of stellar evolution.
Stellar neutrinos can be created through two processes: thermal processes and beta processes. Thermal processes — e.g., pair production, in which a particle/antiparticle pair are created — depend on the temperature and pressure of the stellar core. Beta processes — i.e., when a proton converts to a neutron, or vice versa — are instead linked to the isotopic makeup of the star’s core. This means that, if we can observe them, beta-process neutrinos may be able to tell us about the last steps of stellar nucleosynthesis in a dying star.
But observing these neutrinos is not so easily done. Neutrinos are nearly massless, neutral particles that interact only feebly with matter; out of the whopping ~1060 neutrinos released in a supernova explosion, even the most sensitive detectors only record the passage of just a few. Do we have a chance of detecting the beta-process neutrinos that are released in the final few hours of a star’s life, before the collapse?
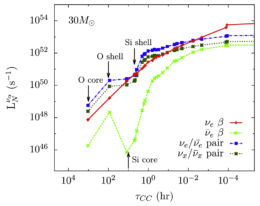
Neutrino luminosities leading up to core collapse. Shortly before collapse, the luminosity of beta-process neutrinos outshines that of any other neutrino flavor or origin. [Adapted from Patton et al. 2017]
Modeling Stellar Cores
To answer this question, Kelly Patton (University of Washington) and collaborators first used a stellar evolution model to explore neutrino production in massive stars. They modeled the evolution of two massive stars — 15 and 30 times the mass of our Sun — from the onset of nuclear fusion to the moment of collapse.
The authors found that in the last few hours before collapse, during which the material in the stars’ cores is rapidly upcycled into heavier elements, the flux from beta-process neutrinos rivals that of thermal neutrinos and even exceeds it at high energies. So now we know there are many beta-process neutrinos — but can we spot them?
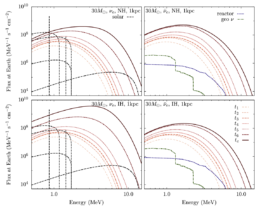
Neutrino and antineutrino fluxes at Earth from the last 2 hours of a 30-solar-mass star’s life compared to the flux from background sources. The rows represent calculations using two different neutrino mass hierarchies. Click to enlarge. [Patton et al. 2017]
Observing Elusive Neutrinos
For an imminent supernova at a distance of 1 kiloparsec, the authors find that the presupernova electron neutrino flux rises above the background noise from the Sun, nuclear reactors, and radioactive decay within the Earth in the final two hours before collapse.
Based on these calculations, current and future neutrino observatories should be able to detect tens of neutrinos from a supernova within 1 kiloparsec, about 30% of which would be beta-process neutrinos. As the distance to the star increases, the time and energy window within which neutrinos can be observed gradually narrows, until it closes for stars at a distance of about 30 kiloparsecs.
Are there any nearby supergiants soon to go supernova so these predictions can be tested? At a distance of only 650 light-years, the red supergiant star Betelgeuse should produce detectable neutrinos when it explodes — an exciting opportunity for astronomers in the far future!
Citation
Kelly M. Patton et al 2017 ApJ 851 6. doi:10.3847/1538-4357/aa95c4
3 Comments
Pingback: capturing a SN's neutrinos
Pingback: Carnaval del espacio # 644 - Universe Today - Enciclopedia Universo
Pingback: Carnaval del espacio # 643 - Universe Today - Enciclopedia Universo