Editor’s note: Astrobites is a graduate-student-run organization that digests astrophysical literature for undergraduate students. As part of the partnership between the AAS and astrobites, we occasionally repost astrobites content here at AAS Nova. We hope you enjoy this post from astrobites; the original can be viewed at astrobites.org!
Title: Models of Warm Jupiter Atmospheres: Observable Signatures of Obliquity
Author: Emily Rauscher
First Author’s Institution: University of Michigan
Status: Published in ApJ
Observing exoplanets is challenging! So how can we ever imagine learning something about their seasons? Over the past decade, astronomers have made extensive progress in understanding the atmospheres of hot Jupiters, including weather detections. But hot Jupiters are tidally locked and therefore experience no seasons. For observations of seasons, we need to push outwards to planets on longer orbits, where tidal interaction with the star is minimal. But a longer orbit means cooler planets. Cooler planets emit less thermal radiation, making them far dimmer than hot Jupiters, with blackbody spectra that peak at longer infrared wavelengths. This population of “warm Jupiters,” or Jupiter-sized planets with temperatures between 500–1000K, are out of reach for current telescopes. But with the James Webb Space Telescope’s 6.5-m mirror and its ability to observe out to longer wavelengths than current telescopes, astronomers will soon be studying the atmospheres of this new population of exoplanets!
How do these warm Jupiters differ from tidally locked hot Jupiters? Gravitational interactions between a star and a planet on a close orbit — like a hot Jupiter — will slow the rotation of the planet to the point where its rotation is the same as its orbital period. As a consequence, one side of the planet always faces its star, while the other side is never illuminated. Tidal locking also circularizes the orbit of a planet and removes any rotational tilt (obliquity). We therefore know the rotational period, eccentricity, and obliquity of a hot Jupiter without any required analysis. Warm Jupiters, on the other hand, are less affected by significant tidal effects, which means we have no intrinsic knowledge of these parameters. The author addresses the obliquity part of this problem in today’s astrobite by posing the following question: can we detect and determine the obliquity of a warm Jupiter and, in doing so, finally observe seasons on an exoplanet?
Wait! Time Out! Obliquities, Rotational Tilt, Seasons?
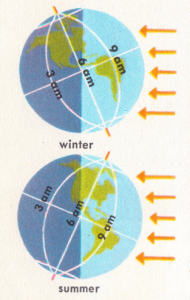
Figure 1: Earth’s rotational or axis tilt is the reason for our season(s). The hemisphere tilted towards the Sun experiences summer while the opposite hemisphere experiences winter. Spring and Fall occur when neither hemisphere is tilted towards the Sun leading to equal heating. [Golden Guide to Weather from St. Martin’s Press]
OK, Got It! Let’s Build a Planet!
The author creates a hypothetical warm Jupiter that has all the same properties of Jupiter, including same radius, mass, and rotational period. But instead of orbiting the Sun once every 5 years, this planet orbits a Sun-like star every 10 days, giving it a temperature of about 900 K. Using a global circulation model (GCM), the author simulates the atmosphere of this warm Jupiter at varying obliquities. What seasons look like on this warm Jupiter is plotted in Figure 2 for obliquities of 30 degrees (top panel), 60 degrees (middle panel), and 90 degrees (bottom panel). The fast rotational period (10 hours) of this planet compared to the orbital period of 10 days causes the atmosphere to smear out most of the day/night temperature contrast, allowing the author to average the temperature over longitude (east–west direction). The larger obliquity correlates with longer and more extreme seasons at higher latitudes (north–south direction). For obliquities greater than 60 degrees, the poles of the warm Jupiter become hotter than the equator, leading to larger temperature contrasts than the 30-degree (Earth-like obliquity) model.
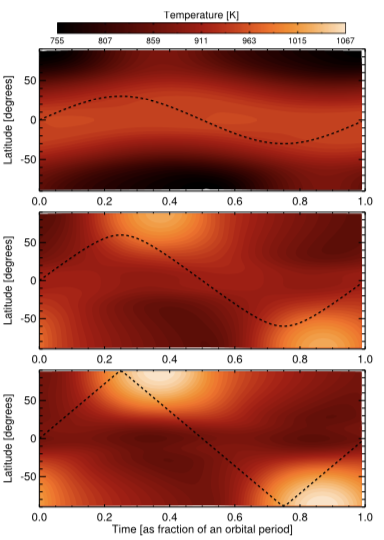
Figure 2: Map of the longitudinally averaged temperature as a function of latitude and time over one orbit. Top panel is a warm Jupiter with 30 degrees obliquity, middle panel is 60 degrees obliquity, and bottom panel has 90 degrees obliquity. The black dashed line represents the location of the subsolar point over time. [Rauscher et al. 2017]
The Planet Now Has Seasons, Let’s “Observe” It
The paper first analyzes the phase curves of these hypothetical warm Jupiters. A phase curve is the light curve of a planet as it orbits around its star. At different points in its orbit, the planet will emit more or less light depending on what fraction of the day side we observe. As the planet has a 10-day orbit, the author notes that this would require continuous observations with JWST for those 10 days. From these phase curve models, the author noticed a degeneracy between the obliquity of a warm Jupiter and its viewing orientation. Figure 3 shows that a planet with the same obliquity can appear very differently depending on from the angle at which we observe it. By summing up the total flux of this planet at different locations in its orbit, we can create phase-curve observations. However, phase curves only provide a 1D total flux map of the planet. Even with the same obliquity, we will observe different amounts of flux simply due to the viewing angle. Phase curves alone don’t provide enough information to measure obliquity and viewing angle independently.
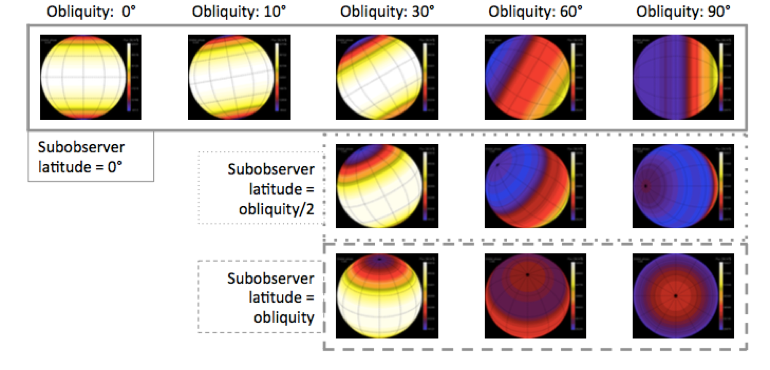
Figure 3: Models of the warm Jupiter at viewed with different orientations. The top panel shows orientations of the planet if we were to observe the planet directly above the equator. The second panel shows the same planet at obliquities 30, 60, and 90 degrees as in the top panel, but twisting the planet towards our line of sight by half of the obliquity value. The bottom panel twists the planet even more towards our line of sight where our viewing angle is equal to that of the planet’s obliquity. For example, the bottom right image twists the planet 90 degrees from its original orientation in the top right image. This adds a complication to the problem, we now have a degeneracy between obliquity and the viewing angle or orientation of the planet to our line of sight. A movie of this figure can be found here. [Rauscher et al. 2017]
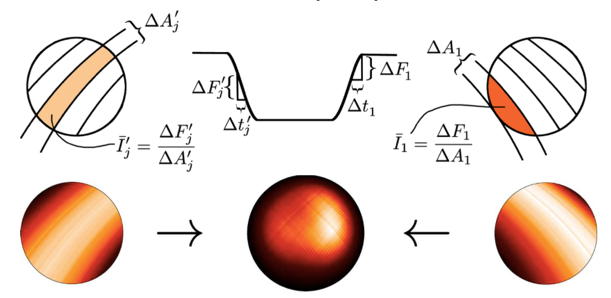
Figure 4: The concept of eclipse mapping. As the planet passes behind its star, slices of the planet map to the slopes of the secondary eclipse. Combining this information with the eclipse depth should help observers distinguish the direction from which we are viewing the planet. [Majeau et al. 2012]
About the author, Jessica Roberts:
I am a graduate student at the University of Colorado, Boulder, where I study extra-solar planets. My research is currently focused on understanding the atmospheres of the extremely low-mass low-density super-puffs. Out of the office, you will probably find me running, cross-stitching, or playing with my dog.
1 Comment
Pingback: are seasons on exoplanets detectable?