Editor’s note: Astrobites is a graduate-student-run organization that digests astrophysical literature for undergraduate students. As part of the partnership between the AAS and astrobites, we occasionally repost astrobites content here at AAS Nova. We hope you enjoy this post from astrobites; the original can be viewed at astrobites.org!
Title: Empirical Determination of Dark Matter Velocities using Metal-Poor Stars
Authors: Jonah Herzog-Arbeitman, Mariangela Lisanti, Piero Madau, Lina Necib
First Author’s Institution: Princeton University
Status: Submitted to ApJL
Our galaxy is embedded in a cloud of dark matter, thought to consist of tiny particles traveling along orbits through the halo. These dark matter particles permeate all regions of the galaxy, extending far beyond the edge of the bright central spiral, but also orbiting through our solar system, and even passing right through the Earth. This is why scientists build giant detectors, hoping to trap some of these dark matter particles as they pass by. So far, these experiments have not detected dark matter, but that lack of detection is actually quite interesting. Finding out what dark matter is not, and thereby narrowing down the possibilities, is an important step towards revealing the true nature of these mysterious particles.
In order to really understand what it means when a detecter does not see dark matter, it is important to have a clear prediction for how much dark matter should be detected. For example, if we expect very few dark matter particles to pass through the Earth in a given amount of time, then maybe the lack of detections over a few years doesn’t actually mean those particles don’t exist. One essential piece of information in this prediction is the velocity of dark matter particles as they orbit past our solar system.
So, how can we determine the speed of these particles that we haven’t even directly detected? Well, let’s look back at where these particles actually come from. Dark matter halos grow over time by consuming other dark matter halos. This process is called hierarchical structure formation. The Milky Way is continuously pulling in smaller galaxies and then tearing them apart, thoroughly mixing their stars and dark matter particles into the Milky Way halo (Figure 1).
This understanding of the origin of these particles reveals an important piece of information: when dark matter particles join the Milky Way, they are often accompanied by stars. This is great news, because stars, unlike dark matter particles, are not invisible, and we can directly measure their velocities. If we can confirm that dark matter particles tend to move at similar velocities to their stellar companions, then this problem of determining the local dark matter velocity is much simpler! Finding out if this is in fact the case is exactly the goal of today’s paper.
The tricky thing here is that the Milky Way is continuously forming new stars, so the authors need to find a way to distinguish between the stars formed within the Milky Way and stars that formed in smaller galaxies and were then consumed by the Milky Way along with the corresponding dark matter. This turns out to be fairly straightforward: stars that form in smaller galaxies tend to have a different chemical composition than stars that are currently being formed in the Milky Way. This is because Milky Way stars are forming from materials that have been enriched with heavier elements by generations of star formation, while the stars in smaller galaxies are not. The stars we are interested in are therefore what astronomers call “metal-poor.” The prediction is therefore that metal-poor stars and dark matter particles should have similar velocities.
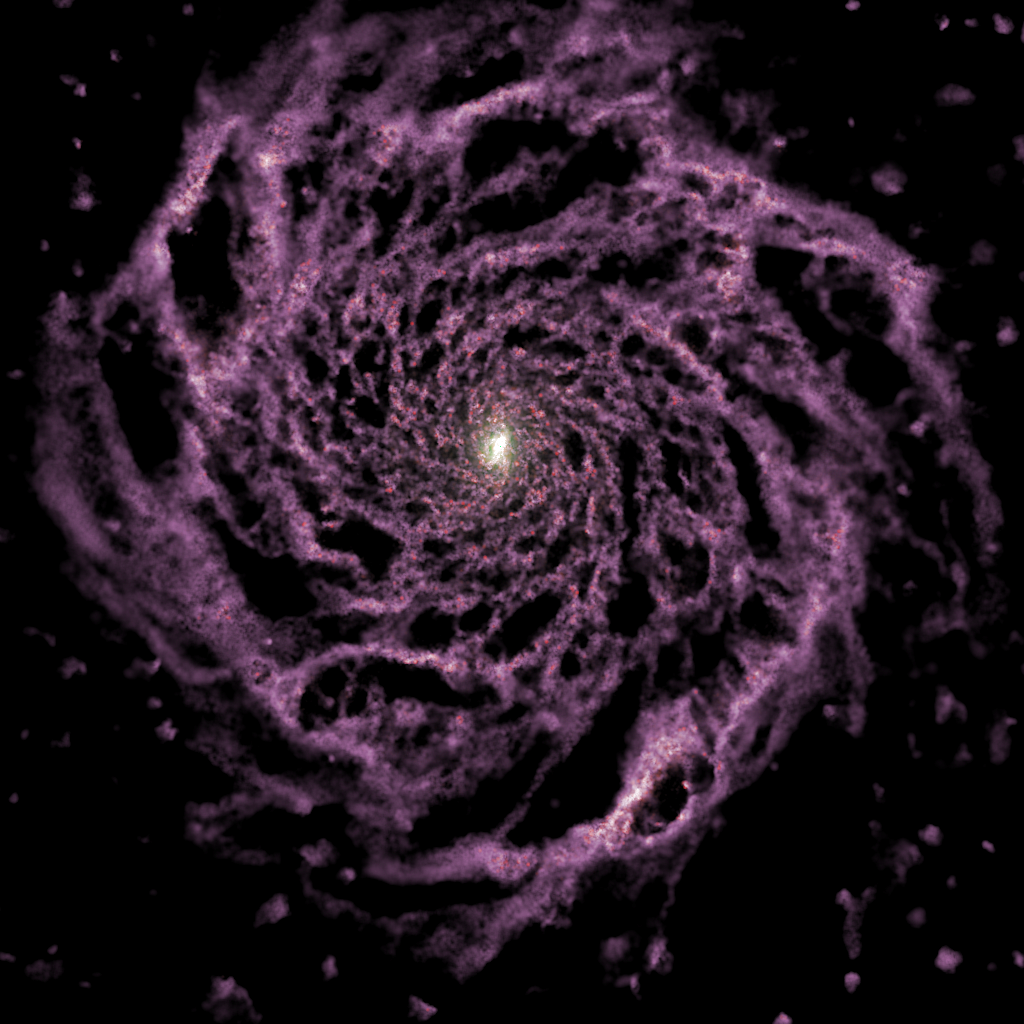
Figure 2. A simulated Milky Way-like galaxy, from the ERIS simulation used in this paper. [Simone Callegari]
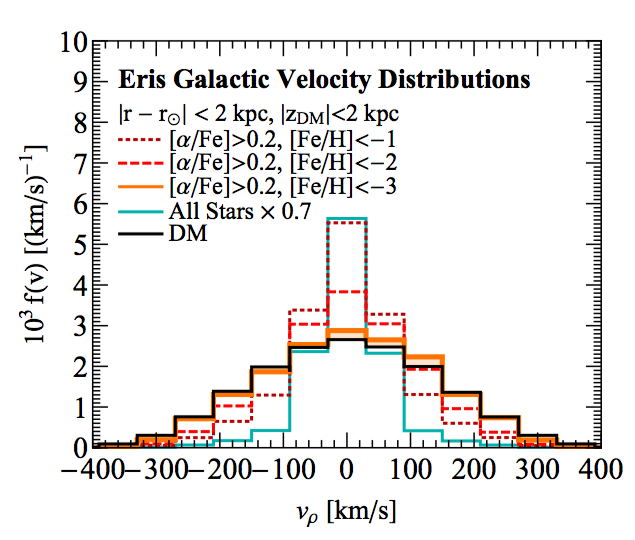
Figure 3. Velocity histograms of different components of the Milky Way, as seen in the ERIS simulation. The black histogram shows the velocity distribution of dark matter. The cyan histogram illustrates the velocity of all stars, and has a much larger central peak than the dark matter distribution. The orange histogram, however, which includes only metal-poor stars, is very similar to the dark matter velocity distribution. [Herzog-Arbeitman et al. 2018]
About the author, Nora Shipp:
I am a 2nd year grad student at the University of Chicago. I work on combining simulations and observations to learn about the Milky Way and dark matter.
1 Comment
Pingback: The speed of dark matter