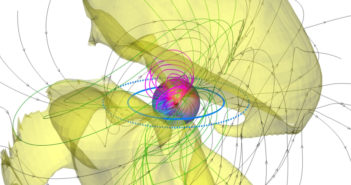
Featured Image: Navigating Turbulence Around an Active Young Star
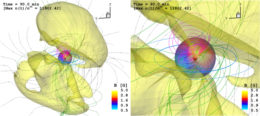
Model results showing the conditions 90 minutes after a coronal mass ejection for a field of view 175 times the stellar radius (left) and 60 times the stellar radius (right). The yellow lobes show the surface on which the plasma density is ten times higher than the typical density. Planetary orbits are shown in blue, and the sphere surrounding the central star shows the magnetic field strength at half the distance to the inner planet. The magenta and green lines show the magnetic fields that loop back to the star’s surface or extend outward, respectively. Click for high-resolution version. [Fraschetti et al. 2022]
Citation
“Stellar Energetic Particle Transport in the Turbulent and CME-disrupted Stellar Wind of AU Microscopii,” Federico Fraschetti et al 2022 ApJ 937 126. doi:10.3847/1538-4357/ac86d7