Editor’s Note: Astrobites is a graduate-student-run organization that digests astrophysical literature for undergraduate students. As part of the partnership between the AAS and astrobites, we occasionally repost astrobites content here at AAS Nova. We hope you enjoy this post from astrobites; the original can be viewed at astrobites.org.
Title: A Detection of Cosmological 21-cm Emission from CHIME in Cross-Correlation with eBOSS Measurements of the Lyα Forest
Authors: CHIME Collaboration
Status: Published in ApJ
A Map of the Universe
Throughout the history of astronomy, we’ve been able to map the universe in a couple of different ways. Large surveys of galaxies, such as the Sloan Digital Sky Survey (SDSS) or the Dark Energy Spectroscopic Instrument survey, are extremely useful — they give astronomers a huge sample of galaxies to characterize, and the positions of those galaxies tell us about how the universe as a whole is set up. At extremely high redshifts, the cosmic microwave background shows us the structure of the universe right as it began. More recently, a totally separate technique has been developing: (spectral) line-intensity mapping. In this technique, you essentially take a very blurry picture of a large region of the universe at a wavelength that corresponds to a specific spectral line. Instead of targeting specific galaxies, you get all of the emission from that spectral line in that region of the universe. This way, you can get light from things that are much fainter than a traditional galaxy survey can see.
The fact that you’re targeting a specific spectral line is also important: because of the expansion of the universe, emission from different distances is redshifted to different observed wavelengths, and so the end product of a line-intensity mapping experiment is a 3D map of the universe instead of just a 2D picture. Because of the expansion of the universe, this also means you map the universe through time. Figure 1 shows a (very idealized) picture of what a single-wavelength slice of this could look like.
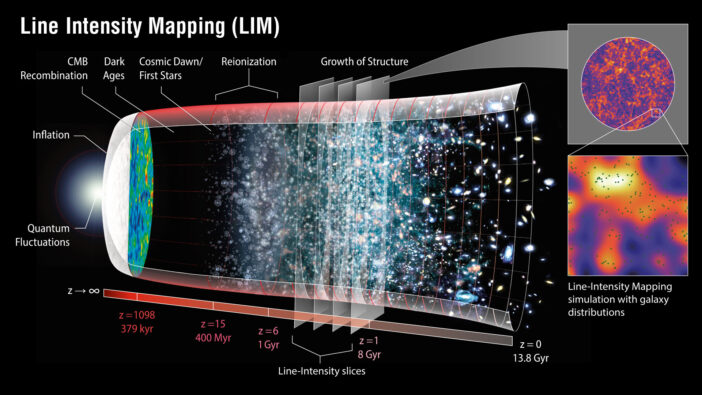
Figure 1: A simulated line-intensity map from a single slice. [NASA / LAMBDA Archive Team]
Neutral About Hydrogen
The line-intensity mapping technique was originally developed for studies of the 21-cm hydrogen line, which is a spin-flip transition of neutral hydrogen mainly emitted by diffuse gas. This is a very powerful technique: it’s extremely difficult to observe neutral hydrogen gas in any other way, and a lot of the universe is made up of this gas, especially at high redshift!
Today’s article is also looking for this 21-cm emission, specifically by using the Canadian Hydrogen Intensity-Mapping Experiment (CHIME; shown in Figure 2). This experiment has been discussed in several astrobites over the past few years, both for its 21-cm work (x) and for its work with fast radio bursts (x, x, x).
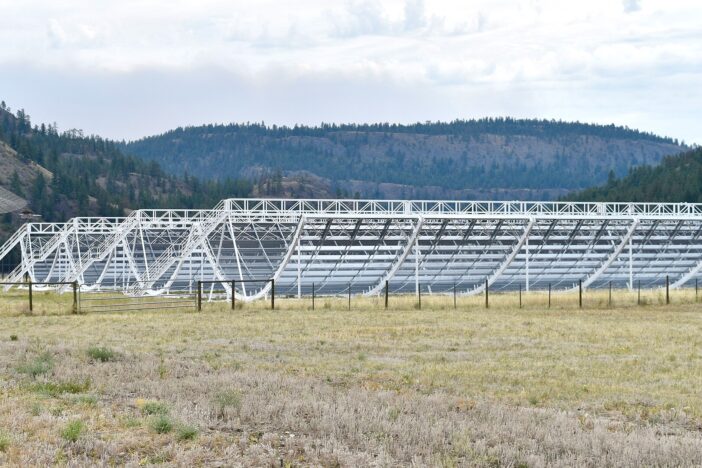
Figure 2: The CHIME instrument. [Wikipedia user Z22; CC BY-SA 4.0]
Fighting Foregrounds with Friends
Detecting 21-cm emission is significantly complicated by the fact that there are lots of things in the way! Between Earth and the distant galaxies astronomers are actually after, there are many other sources that emit around a wavelength of 21 cm, including Earth’s ionosphere and synchrotron emission from the Milky Way. These are “foregrounds,” and they’re far brighter than the 21-cm emission astronomers are looking for! Although you can work around foregrounds using 21-cm data alone, combining your 21-cm measurement with other measurements of the structure you’re trying to see (taken using other wavelengths of light) makes for a much more robust approach. Because the two measurements are taken using different wavelengths, it’s very unlikely that they’ll show the same foreground structure. When you use a statistical technique such as cross-correlation to combine the two, the foregrounds (more or less) disappear! This has already been done for the CHIME 21-cm data at low redshifts, but not at redshifts above z = 1.5 (9 billion years ago).
In this research article, the authors combine their 21-cm data with Lyα forest measurements from the extended Baryonic Oscillation Spectroscopic Survey (eBOSS), which is a part of SDSS. Importantly, the Lyα forest traces absorbing hydrogen gas, and the 21-cm data trace emitting hydrogen gas. Density determines whether gas absorbs or emits: denser gas tends to absorb radiation, and more diffuse gas tends to emit radiation. This means that on small scales, the two signals are actually expected to be anti-correlated (because the measurements are coming from different kinds of gas), and the cross-correlation signal will be negative.
A Negative Detection!
Indeed, after reducing the CHIME 21-cm data, the authors do detect anti-correlation! Figure 3 shows the cross-correlation signal. This is measured by introducing an artificial offset in the x-axis (in this case, the frequency axis) between the 21-cm measurement and the Lyα forest measurement, and then measuring how much these two signals correlate or anti-correlate at that offset. The amount of correlation is then shown as a function of this frequency offset. You can see a large negative spike right at zero offset, where the two signals should anti-correlate the most. The dotted black line shows a model the authors came up with for this cross-correlation, and the two agree quite well (the bottom panel shows the residuals between the signal and the model).
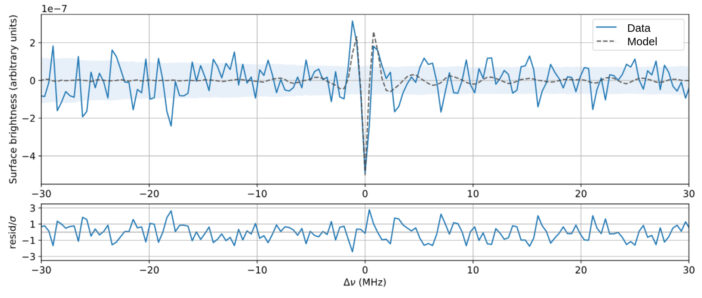
Figure 3: Top: The cross-correlation signal shown as a function of offset between the two datasets in the frequency axis. The blue line is the actual CHIME x eBOSS data, and the dotted black line is a model of what the signal should look like, fit to the data in amplitude but nothing else. Bottom: The residuals between the data and the model, expressed in terms of their significance. [Adapted from CHIME Collaboration 2024]
Original astrobite edited by Nathalie Korhonen Cuestas.
About the author, Delaney Dunne:
I’m a PhD student at Caltech, where I study how galaxies form and evolve by mapping their molecular gas! I do this using COMAP, a radio-frequency line-intensity mapping experiment based in California’s Owens Valley.