The realm of high-energy astrophysics is populated with extreme objects like accreting supermassive black holes and exploding stars. Among the most intriguing objects in the high-energy category are pulsars and magnetars, both of which fall under the umbrella of neutron stars: extremely dense, city-sized remnants of collapsed massive stars.
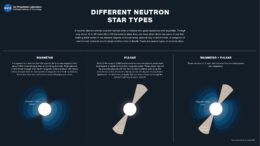
Infographic describing some of the characteristics of pulsars and magnetars. Click to enlarge. [NASA/JPL-Caltech]
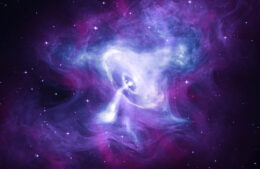
A multiwavelength image of the center of the Crab Nebula supernova remnant, showing the distinct jet and torus of the pulsar wind nebula. [X-ray: NASA/CXC/SAO; Optical: NASA/STScI; Infrared: NASA-JPL-Caltech]
Tracing a Pulsar Wind Nebula
More than a decade ago, X-ray observations revealed the presence of an energetic pulsar, PSR J1849-0001, and its surrounding pulsar wind nebula: a glowing cloud energized by the high-energy charged particles shed by a young pulsar as it spins down. Pulsar wind nebulae are sometimes found at the centers of supernova remnants, as is the case for the famous Crab Nebula.
Seth Gagnon (George Washington University) and coauthors used the Chandra X-ray Observatory to study PSR J1849-0001 and its surrounding pulsar wind nebula. The observations showed that the structure of the nebula isn’t well defined, lacking the distinct jet and torus regions of other pulsar wind nebulae. PSR J1849-0001’s nebula does exhibit a faint jet, making it similar in appearance to the pulsar wind nebula of another young pulsar, J1811-1925. The team concluded that the angle between the pulsar’s spin and magnetic axes is likely small, potentially placing it in the class of “MeV pulsars” that emit pulses of high-energy X-rays.
In addition to illuminating the amorphous nature of the nebula, the team’s Chandra observations also picked up bright X-ray emission from a pair of stars that orbit each other widely. Given the stars’ wide separations, it’s not clear what kind of interaction could be powering the observed bright and variable X-ray emission — leaving a mystery to be solved by future observations!Tracking a Magnetar
Roughly 20% of known and candidate magnetars have been observed at radio wavelengths, emitting pulses of radio emission that are distinct from the signals from pulsars. Hao Ding (National Astronomical Observatory of Japan) and collaborators used the Very Long Baseline Array to monitor the radio emissions of Swift J1818.0−1607, the fastest-spinning and youngest magnetar known.
Why monitor a magnetar? The formation mechanism for these extreme objects isn’t yet known, though several of them are thought to be associated with supernova remnants, making core-collapse supernovae their likely origin. One way to test different formation theories is by measuring how quickly magnetars move through space, as different origin stories impart “kicks” of different strengths on a newborn magnetar. Ding’s team used their three-year observing campaign to determine J1818.0−1607’s parallax and proper motion, which together yielded the magnetar’s distance and velocity perpendicular to our line of sight.
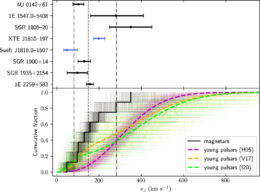
Top: Transverse space velocities of eight magnetars. Bottom: Cumulative distribution of transverse velocities of magnetars and young pulsars. Click to enlarge. [Ding et al. 2024]
As for the origins of J1818.0−1607, the team found that a radio-emitting shell of gas that was previously discovered nearby is located at roughly the same distance as the magnetar, strengthening the possibility that this shell is a supernova remnant.
Pulsars: Not Just Radio Sources
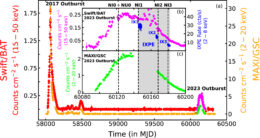
The timeline of the IXPE, NICER, and NuSTAR observations along with observations from the Swift Observatory Burst Alert Telescope (BAT) and the Monitor of All-sky X-ray Image (MAXI). NuSTAR and NICER observations are marked by the vertical dashed lines. The IXPE measurements are marked with blue circles. Click to enlarge. [Majumder et al. 2024]
The IXPE observations provided the first measurement of this pulsar’s X-ray polarization, or the orientation of its light waves as they travel through space. Like other X-ray pulsars monitored with IXPE, J0243.6+6124’s X-ray emission is weakly polarized (polarization fraction of ~2–3%) compared to what models predict. Based on the current IXPE, NuSTAR, and NICER data, Majumder’s team suggests that the unexpectedly low polarization fraction of J0243.6+6124 is due to vacuum resonance at the interface between the pulsar’s crust and interior, though other mechanisms are possible.
Millisecond Monitoring
The final study of this Monthly Roundup concerns millisecond pulsars. All pulsars spin incredibly fast, but millisecond pulsars are the fastest of the fast, with spin periods less than about 10 milliseconds. Although pulsars tend to spin more slowly as they age, researchers believe that millisecond pulsars buck this trend. Instead, millisecond pulsars are likely older pulsars that have been “spun up” to high speeds by accreting matter from a close binary companion. While many millisecond pulsars have been found to have binary companions, some of them fly solo, and the formation mechanism of these single millisecond pulsars isn’t yet clear. Regardless of whether they are singletons or in pairs, more observations are needed to characterize these extreme objects.
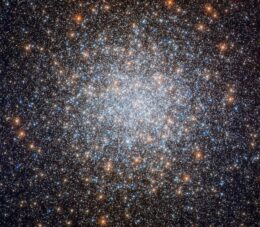
Hubble Space Telescope image of the globular cluster Messier 3. Messier 3 is home to 500,000 stars and six known millisecond pulsars. It’s also the first Messier object to have been discovered by Charles Messier himself. [ESA/Hubble & NASA, G. Piotto et al.; CC BY 4.0]
For the remaining five pulsars, Li’s team obtained updated or new timing solutions: models of a pulsar’s pulses that can be used to predict future pulse arrival times. Additionally, they showed for the first time that pulsars M3E and M3F have low-mass companion objects and travel on circular orbits of 7.1 and 3.0 days, respectively.
In future work, Li’s team plans to focus on scintillation; while scintillation can be an annoyance to researchers when it disperses pulsar signals, it also provides a way to study the intervening interstellar material.
Citation
“Chandra X-Ray Observations of PSR J1849-0001, Its Pulsar Wind Nebula, and the TeV Source HESS J1849-000,” Seth Gagnon et al 2024 ApJ 968 67. doi:10.3847/1538-4357/ad3e6d
“VLBA Astrometry of the Fastest-Spinning Magnetar Swift J1818.0−1607: A Large Trigonometric Distance and a Small Transverse Velocity,” Hao Ding et al 2024 ApJL 971 L13. doi:10.3847/2041-8213/ad5550
“First Detection of X-Ray Polarization in Galactic Ultraluminous X-Ray Pulsar Swift J0243.6+6124 with IXPE,” Seshadri Majumder et al 2024 ApJL 971 L21. doi:10.3847/2041-8213/ad67e5
“Timing and Scintillation Studies of Pulsars in Globular Cluster M3 (NGC 5272) with FAST,” Baoda Li et al 2024 ApJ 972 43. doi:10.3847/1538-4357/ad5a82