Choosing a favorite planet has never been more challenging: researchers have discovered more than 5,600 exoplanets that range from super-heated super-puffs skimming the surfaces of their host stars to Mercurian worlds of pure iron. As telescopes dedicate even more time to the search for distant worlds, the exoplanets discovered are getting smaller, cooler, and more like Earth. The allure of temperate, Earth-like exoplanets is undeniable, but so are the roadblocks to finding and characterizing these planets. In today’s Monthly Roundup, we’ll give an overview of four recent studies of temperate exoplanets, ranging from ways to detect technological civilizations on these worlds to the importance of fine-tuning model inputs.
Temperate Earth-Sized Planet Discovered
The first of today’s articles examines a potentially Earth-like planet orbiting an M dwarf — the smallest, coolest, and most common type of star in our galaxy. The star in question, Gliese 12, is about a quarter of the mass of the Sun, has a temperature around 3000K, and sits just 40 light-years away. In 2023, the Transiting Exoplanet Survey Satellite (TESS) spotted a planet passing in front of the star but couldn’t pin down its orbital period. Now, a team led by Masayuki Kuzuhara (National Institute of Natural Sciences, Japan) and Akihiko Fukui (University of Tokyo and Institute of Astrophysics of the Canary Islands) has analyzed follow-up observations to characterize this nearby world.The team found that Gliese 12 b swings around its host star every 12.76 days and is just a hair smaller than Earth. Its mass might be far greater, though, with a tentative upper limit of 3.9 Earth masses. The large mass range means the planet could be extraordinarily dense — nearly as dense as pure iron — or it could be rocky with a lightweight hydrogen atmosphere. The planet’s equilibrium temperature is a relatively cool 315K (107℉/42℃), but if this planet is blanketed by a thick, Venus-like atmosphere, its true surface temperature could be far hotter.
This discovery is significant because most of the exoplanets known to orbit M-dwarf stars are fried by high-energy radiation, making them inhospitable to life. Unlike many M dwarfs, Gliese 12 appears to be inactive and unlikely to unleash planet-searing flares. Thus, Gliese 12 b joins the short list of nearby temperate exoplanets whose atmospheres could be scrutinized with JWST, and future observations should reveal more about this world.
Inputs Under Investigation
Let’s say you’ve discovered a temperate exoplanet and collected a spectrum of its atmosphere. What happens next? Researchers rely on complex models to interpret their observations of planetary atmospheres. Models of exoplanet atmospheres take in myriad inputs, and tweaking any one of these inputs can have significant impact on the output, as recent research led by Wynter Broussard (University of California, Riverside) shows.
Broussard’s team tested the impact of varying the water photolysis cross section on models of temperate planets without oxygen and without life. Photolysis is the splitting apart of a molecule by a photon; the photolysis cross section describes how readily a molecule is broken apart by a photon of a given wavelength. When a photon cleaves apart a water molecule, the interaction leaves behind a hydrogen atom and a radical, OH. OH is highly reactive and can destroy trace atmospheric gases. Because of this, the photolysis cross section of water molecules has a potentially large impact on the predicted composition of a planet’s atmosphere.
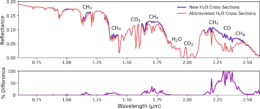
Modeled spectra of an exoplanet orbiting a Sun-like star with the new water cross section (purple) and the old water cross section (coral). Click to enlarge. [Broussard et al. 2024]
Signals from Sulfur on Water-World Exoplanets
Our ability to interpret atmospheric spectra is critical: it might be our best hope of detecting life from light-years away. In September 2023, the temperate sub-Neptune planet K2-18b made a splash when researchers announced that it’s likely an ocean-covered world whose atmosphere contains methane, carbon dioxide, and maybe, tentatively dimethyl sulfide, which on Earth is only produced by life. Subsequent research suggested K2-18b fits the model of a lifeless, gas-rich planet equally well, highlighting the difficulty of this type of research.
Now, a team led by Shang-Min Tsai (University of California, Riverside) has examined the chemistry and detectability of sulfur-containing gases — like dimethyl sulfide — that are produced by life. Tsai and collaborators simulated the atmospheres of sub-Neptune-sized water-world exoplanets to understand how sulfur compounds might circulate within a planet’s atmosphere and be detectable through transmission spectroscopy.
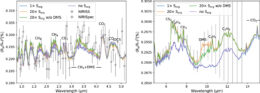
Modeled transmission spectra of K2-18b containing the same flux of sulfur produced by life as Earth (blue), 20 times the biogenic sulfur of Earth (orange), and 20 times the biogenic sulfur of Earth but with the signal from dimethyl sulfide removed (green). The purple line shows the spectrum of a planet with no biogenic sulfur. Click to enlarge. [Tsai et al. 2024]
From Biosignatures to Technosignatures
The final article of this Roundup examines a way to find intelligent life. Searches for intelligent life beyond Earth focus on technosignatures, or signs of technology, like radio signals, enormous structures created to capture starlight, or atmospheric gases from industrial processes.
Edward Schwieterman (University of California, Riverside, and Blue Marble Space Institute of Science) and collaborators considered the possibility of detecting artificial greenhouse gases injected into a planet’s atmosphere to alter its climate, such as to stave off an oncoming ice age or to make a chilly neighboring world more hospitable. The gases studied in this work — hexafluoroethane (C2F6), octafluoropropane (C3F8), sulfur hexafluoride (SF6), nitrogen trifluoride (NF3), and carbon tetrafluoride (CF4) — are unlikely to be produced by non-technologically advanced life and may linger in planetary atmospheres for millennia, increasing the odds that we could detect these gases.
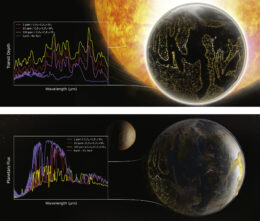
Illustration of modeled transmission (top) and emission (bottom) spectra from a hypothetical Earth-like exoplanet whose atmosphere contains a combination of three technosignature gases: C3F8, C2F6, and SF6. Click to enlarge. [Schwieterman et al. 2024]
Citation
“Gliese 12 b: A Temperate Earth-Sized Planet at 12 pc Ideal for Atmospheric Transmission Spectroscopy,” Masayuki Kuzuhara et al 2024 ApJL 967 L21. doi:10.3847/2041-8213/ad3642
“The Impact of Extended H2O Cross Sections on Temperate Anoxic Planet Atmospheres: Implications for Spectral Characterization of Habitable Worlds,” Wynter Broussard et al 2024 ApJ 967 114. doi:10.3847/1538-4357/ad3a65
“Biogenic Sulfur Gases as Biosignatures on Temperate Sub-Neptune Waterworlds,” Shang-Min Tsai et al 2024 ApJL 966 L24. doi:10.3847/2041-8213/ad3801
“Artificial Greenhouse Gases as Exoplanet Technosignatures,” Edward W. Schwieterman et al 2024 ApJ 969 20. doi:10.3847/1538-4357/ad4ce8