A supernova is a spectacular way for a star to die. Massive stars meet this fate when the outward pressure exerted by core nuclear fusion can no longer hold off the gravity of the star’s outer layers, and the remnants of lower-mass stars can attain this honor through accretion or collisions. Today we’ll introduce four research articles that examine various aspects of supernova science, from attempts to determine how lightweight a supernova progenitor star can be to exploring why some massive stars don’t produce supernovae at all.
Probing the Smallest Supernova Stars
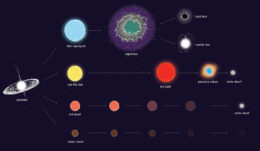
The main stellar evolution pathways. It’s not yet clear exactly which stars explode as supernovae and which become white dwarfs. Click to enlarge. [ESA]
Luckily, increasing coverage by transient-hunting surveys has generated a growing sample of these faint, fast events. Kaustav Das (California Institute of Technology) and coauthors studied nine supernovae detected by the Zwicky Transient Facility that were found to have certain chemical abundance ratios that hint at the progenitor stars being low mass. These supernovae are calcium-rich Type IIb supernovae, which have much larger [Ca II]/[O I] ratios than typical core-collapse supernovae.
Das’s team used spectra of each of these supernovae to measure the amount of oxygen present, which can be used in theoretical models to estimate the mass of the star that exploded. The mass estimates for all stars in the sample were less than 12 solar masses, suggesting that this type of supernova tends to arise from stars near the low-mass end of the progenitor mass range. The current sample of known calcium-rich Type IIb supernovae is still small, but future detections should allow researchers to refine models and improve estimates.
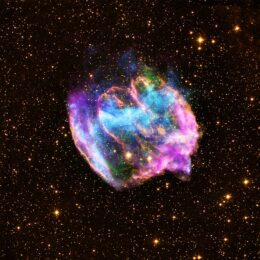
Not all massive stars end their lives as supernovae, leaving behind a supernova remnant like W49B shown here. [X-ray: NASA/CXC/MIT/L.Lopez et al.; Infrared: Palomar; Radio: NSF/NRAO/VLA]
Focusing on Failed Supernovae
When massive stars extinguish their core nuclear fusion and collapse, do they always generate luminous supernovae? Both observations and theory suggest that the answer is no, with some would-be supernovae forming a black hole with no accompanying supernova. Up to a third of massive stars might fail to generate a supernova!
Eric Coughlin (Syracuse University) performed a mathematical exploration of failed supernovae, focusing on the creation and propagation of a shock between the collapsing core and the outward-moving outer layers of the star. Coughlin showed that while some dying massive stars don’t produce supernovae per se, they still undergo an explosion that marks their impending demise. The strength of the explosion from a failed supernova depends on the properties of the star and how much of its mass is lost in the form of neutrinos: chargeless, nearly massless subatomic particles that rarely interact with matter.
In addition to the mathematical solutions that described the explosions, the equations also permitted a solution in which the matter settles near the central object. While we’ll have to wait for future work for a full examination of these solutions, it’s possible that they’ll apply to smaller stellar outbursts that do not destroy the star.
Prospects for Detecting Supernova Neutrinos
When a massive star’s core collapses, it can form a black hole or a neutron star: an extremely dense, rapidly spinning, city-sized sphere made almost entirely of neutrons. As protons and electrons are crushed into neutrons in the star’s core, the transformation produces neutrinos that push the star’s collapsing outer layers outward. While most of the star’s outer layers escape, forming the glowing, complex structures of a supernova remnant, a small fraction of the material falls back onto the protoneutron star, generating even more neutrinos.
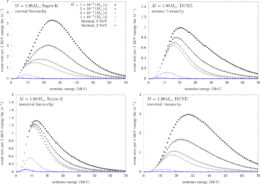
The event rates for a 1.98-solar-mass protoneutron star with various accretion rates as seen by Super-Kamiokande (left) and DUNE (right) and for normal (top) and inverted (bottom) neutrino mass hierarchies. [Akaho et al. 2024]
Akaho’s team found that the mass of the protoneutron star and the rate at which it gathers material from its surroundings both have an impact on the output neutrino luminosity and the average energy of the neutrinos. For a supernova happening about 33,000 light-years away, the neutrino flux should rise above the background measured by the existing Super-Kamiokande and under-construction Deep Underground Neutrino Experiment (DUNE) detectors. The exact strength of the signal depends on several factors, including neutrino oscillation — the process through which a neutrino born in a certain “flavor” morphs to a different flavor as it travels through space.
Investigating Type Ia Supernova Diversity
Supernovae aren’t always the result of massive stars collapsing. Many arise from white dwarfs, which are the exposed cores of low- to intermediate-mass stars that have finished fusing hydrogen in their cores and lost their outer layers. When a white dwarf accretes gas from a companion star, the white dwarf gains mass and heats up, eventually triggering a supernova. Alternatively, the collision of two white dwarfs can generate a supernova. Supernovae arising from white dwarfs are called Type Ia or thermonuclear runaway supernovae.
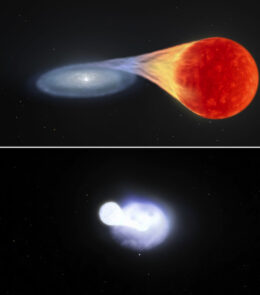
Illustrations of the two main Type Ia supernova pathways: the single-degenerate model (top) and the double-degenerate model (bottom). [Both images from NASA’s Goddard Space Flight Center Conceptual Image Lab]
The team selected a sample of 14 Type Ia supernovae for which spectra were collected within one week of the explosion being detected at Earth. The sample included high-velocity supernovae, normal-velocity supernovae, and some that were similar to the peculiar supernova SN 1999aa. The team then used radiative transfer modeling to model the spectra and extract the properties of the supernova ejecta. Ultimately, they found that the supernovae fell into two groups: one with high-density, carbon-poor ejecta, which makes up the high-velocity sample and some of the normal-velocity sample and one that has low-density, carbon rich ejecta, which makes up the remaining normal-velocity sample and those like SN 1999aa. While more work remains to be done, the team suspects these two groups might be the result of different formation mechanisms.
Citation
“Probing the Low-Mass End of Core-Collapse Supernovae Using a Sample of Strongly Stripped Calcium-Rich Type IIb Supernovae from the Zwicky Transient Facility,” Kaustav K. Das et al 2023 ApJ 959 12. doi:10.3847/1538-4357/acfeeb
“The Division Between Weak and Strong Explosions from Failed Supernovae,” Eric R Coughlin 2023 ApJ 955 110. doi:10.3847/1538-4357/acf313
“Detectability of Late-Time Supernova Neutrinos with Fallback Accretion onto Protoneutron Star,” Ryuichiro Akaho et al 2024 ApJ 960 116. doi:10.3847/1538-4357/ad118c
“Systematic Investigation of Very-early-phase Spectra of Type Ia Supernovae,” Mao Ogawa et al 2023 ApJ 955 49. doi:10.3847/1538-4357/acec74