Gravitational waves from colliding neutron stars have improved our understanding of the interiors of these fantastically compressed objects and helped us measure their radii. How much more precisely will we be able to measure neutron stars with future gravitational wave observatories?
Measuring a Neutron Star
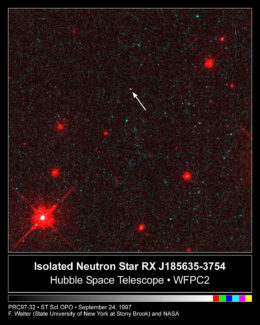
This image from the Hubble Space Telescope shows a neutron star. It was estimated to be no more than 28 kilometers (16.8 miles) across and have a temperature of 1,200,000℉ (670,000℃). [Fred Walter (State University of New York at Stony Brook) and NASA/ESA; CC BY 4.0]
Already, observations of gravitational waves from colliding neutron stars have helped us hone our estimates of the neutron star equation of state. A 1.4-solar-mass neutron star — around the lower limit of a neutron star’s mass — will have a radius between 10.5 and 13 kilometers. Researchers suspect that future gravitational wave observations will narrow this range further, and new work explores how precisely we’ll be able to measure neutron stars in the future.
Computing Collisions
To probe this question, Daniel Finstad (University of Washington; University of California, Berkeley; Lawrence Berkeley National Laboratory) and collaborators Laurel White and Duncan Brown (both Syracuse University) simulated the gravitational waves produced by many pairs of colliding neutron stars.
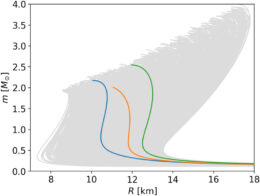
Soft, medium, and stiff equations of state (blue, orange, and green lines, respectively), as well as the full set of equations of state used in the analysis. [Finstad et al. 2023]
Upcoming Observations
With a suite of simulations in hand, Finstad’s team modeled what future gravitational wave observatories would detect if faced with these synthetic signals. They considered future upgrades to the Laser Interferometer Gravitational-Wave Observatory (LIGO) and Virgo detectors that would bring them up to their maximum sensitivity as well as the proposed Cosmic Explorer, which would have arms 10 times as long as LIGO’s and therefore be more sensitive.
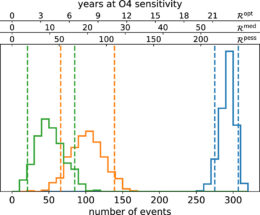
Number of years needed for LIGO–Virgo to observe enough mergers to measure the neutron star equation of state to a precision of 2%. Results are shown for stiff, medium, and soft equations of state (green, orange, and blue, respectively), as well as for different values for the neutron star merger rate, shown with the timescales at the top. [Finstad et al. 2023]
Citation
“Prospects for a Precise Equation of State Measurement from Advanced LIGO and Cosmic Explorer,” Daniel Finstad et al 2023 ApJ 955 45. doi:10.3847/1538-4357/acf12f