Solar flares are bursts of high-energy radiation that are associated with sunspots. New research uses models to study what happens when sunspots collide and under what conditions these collisions cause solar flares.
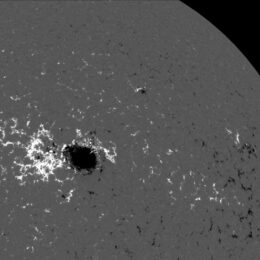
The color scale in this image shows the strength of the radial magnetic field, with outward-pointing fields in white and inward-pointing fields in black. There is a large sunspot on the left side of the image. [NASA/SDO]
Critical Spots for Research
Sunspots are relatively cool areas of the Sun’s disk (3000–4000K as compared to the 5800K surface) where the solar magnetic field rises above the surface. Sunspots often come in pairs, with one found where magnetic field lines emerge from the solar surface and the other where the field lines plunge back in. When the inward- and outward-pointing magnetic fields of two sunspots meet, the release of magnetic energy can power solar flares and other explosive events.
Not all sunspot collisions produce solar flares, though, and understanding why is a goal of solar physics research. Now, researchers have used fluid dynamics simulations to understand the complex magnetic environment surrounding clashing sunspots.
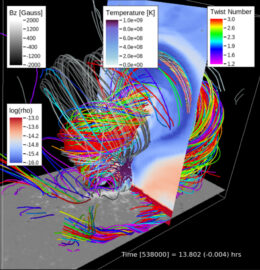
Simulation snapshot showing the magnetic field configuration 14 seconds before the peak of the flare. [Adapted from Rempel et al. 2023]
When Sunspots Collide
Using the three-dimensional MURaM hydrodynamics model, Matthias Rempel (National Center for Atmospheric Research) and collaborators placed sunspots on a collision course, varying the speed of the sunspots and their distance apart to understand how these factors affect the production of solar flares. They started the sunspots at 25,000 kilometers apart, and the programmed trajectories brought the spots as close as 8,500 kilometers. As the sunspots approached and moved past each other, the moving spot broke apart in a process called collisional shearing. This shearing process also occurs in real sunspots, and it may be important to the creation of solar flares.
All of the collisions heated up the solar atmosphere above the sunspots, but only certain simulations resulted in solar flares and ejections of plasma from the solar atmosphere. The higher the velocities of the sunspots and the closer they got together, the more energy was produced, with the distance of closest approach being the biggest determinant of whether or not a solar flare occurred. The team also found that when solar flares did happen, they made good use of the available magnetic energy; 40–50% of the built-up magnetic energy was released in the flares.
Drawing a Line on the Sun
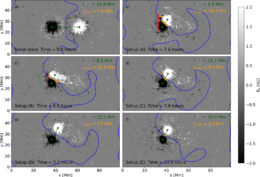
Magnetic fields during the initial state (top left) and closest encounters (remaining panels). The orange lines show the polarity inversion lines. Click to enlarge. [Rempel et al. 2023]
The simulated solar flares in this study reached energies corresponding to M-class flares, the second most energetic class of solar flares. Rempel’s team suspects that by cranking up the magnetic flux to the values seen in highly active sunspots, even more powerful flares are possible.
Citation
“Comprehensive Radiative MHD Simulations of Eruptive Flares Above Collisional Polarity Inversion Lines,” Matthias Rempel et al 2023 ApJ 955 105. doi:10.3847/1538-4357/aced4d