Editor’s Note: This week we’ll be writing updates on selected events at the 55th Division for Planetary Sciences (DPS) meeting, held jointly with the Europlanet Science Congress (EPSC) in San Antonio, Texas, and online. The usual posting schedule for AAS Nova will resume on October 9th.
Table of Contents:
- Alexander Prize Lecture: Results from Monitoring the Giant Planets with Hubble (Amy Simon)
- Round Table Discussion: The Search for Liquid Water beneath the Martian South Polar Layered Deposits (Roberto Orosei, David Stillman, Ali Bramson, and Jack Holt)
Alexander Prize Lecture: Results from Monitoring the Giant Planets with Hubble (Amy Simon)
The Claudia J. Alexander Prize is awarded to a mid-career planetary scientist “who has made and continues to make outstanding contributions that have significantly advanced our knowledge of planetary systems, including our solar system.” This year’s award went to Amy Simon (NASA Goddard Space Flight Center), who described in today’s plenary lecture an ongoing Hubble Space Telescope program to monitor the four giant planets in our solar system.
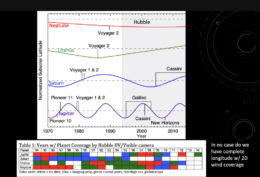
Illustration of the inconsistent observing history of the giant planets. Click to enlarge. [Slide by Amy Simon]
The solution? The Outer Planet Atmospheres Legacy (OPAL) program, which began in 2014 and continues to monitor each of the four giant planets on an annual basis using the Hubble Space Telescope. Each year, Hubble monitors the four planets through two planetary rotations, and the data become immediately available to the public, with the global maps having a dedicated page on the Hubble archive. These observations have allowed us to study storms on Saturn, Saturn’s color changes and changes to its north polar hexagon, the evolution of polar hazes on Uranus, the emergence of new dark spots on Neptune, and much more.
While the current program is scheduled to continue as long as Hubble is able to make the observations, Simon already has an eye on JWST’s infrared observing capabilities. Speaking about the possibility of undertaking a similar observing campaign with JWST, Simon says simply, “Why not?”Round Table Discussion: The Search for Liquid Water Beneath the Martian South Polar Layered Deposits (Roberto Orosei, David Stillman, Ali Bramson, and Jack Holt)
The final plenary session of the meeting was a discussion of an ongoing debate in the planetary science community: whether radar observations show the presence of liquid water beneath Mars’s south polar ice cap. Numerous research articles have argued both sides of the issue, and today four scientists presented their arguments before opening the floor to discussion.
First, a quick primer on the technique used to make the contested measurements. The supposed detection of liquid water was made using radar subsurface sounding, which can peer beneath the surface of a planet. The radar signal is reflected when the electric permittivity (related to how a material responds when exposed to an electric field) changes, such as where a layer of rock meets a layer of ice. How long it takes the signal to return and the brightness of the return signal can be used to map the layers below the surface.
Roberto Orosei (Italian National Institute of Astrophysics) and David Stillman (Southwest Research Institute) presented their evidence for the presence of liquid water first. They noted that the larger the contrast in permittivity of two materials, the stronger the radar reflection will be at their interface. Water or brine (a mixture of water and salts) has a permittivity around 80, while dry materials like rock have permittivities in the 3–15 range. Mixing water into other materials, such as Martian surface material or regolith, also causes a sharp increase in permittivity.
When the team observed strong radar reflections beneath the south polar layered deposits on Mars, liquid water was an obvious candidate. The team observed the strong radar echoes over many different spacecraft orbits, during different seasons, and at different frequencies, showing that the signal is not a fluke. Analysis of the signal suggested that the permittivity of the material is greater than 20 — far too large for a dry material. Orosei and Stillman propose that water mixed with salts and sediment, which would have a lower freezing/melting point, is the cause. Furthermore, research has found that the radar echo properties are similar to those of subglacial lakes on Earth.Orosei and Stillman addressed four common rebuttals of their argument:
- It is too cold beneath the polar deposits for water or brines to be liquid … but brines can be liquid down to 197K, which could be cool enough for them to be liquid in the extreme cold beneath the polar cap, according to the weakening of the radar signal that suggests temperatures below 230K.
- Geologic evidence doesn’t support the presence of a body of water, and the bright radar reflections don’t overlap with the expected locations of lakes … but our data don’t have fine enough vertical resolution to truly determine where lakes should be located beneath the glacier, and the surface features are consistent with what we see above subglacial lakes on Earth.
- Other materials like clays, saline ices, hematite, and smectites can have equally bright radar reflections … but measurements of the permittivities of some of these materials at cold, Mars-like temperatures are too low to explain the bright reflections, and you’d need an unreasonably large amount of hematite to create a similar radar echo. Radar echoes from dense basalts are a possibility.
- The echoes could be an electromagnetic artifact … but looking at raw data or frequency-dependent behavior should rule out this possibility, and the team doesn’t claim that every single bright radar echo is evidence of water.
Next, Ali Bramson (Purdue University) and Jack Holt (University of Arizona Lunar and Planetary Laboratory) took to the stage to present their arguments. First, Bramson outlined how much heat it would take (in the form of geothermal energy radiating out of Mars’s surface) for liquid water to be present beneath the north polar region. The expected heat flux is 10–30 milliwatts per square meter, which could bring temperatures up to 170K, but liquid water with a melting point of 273K would require at least 200 milliwatts per square meter. Even with salts mixed in to the ice, the melting point hovers around 200K, which still requires an increased amount of heating. Bramson and collaborators investigated possible ways to increase the geothermal heat flux, settling on magmatic activity within the past few hundred thousand years as a possibility. However, past magmatic activity is likely to be ancient, and most signs of “recent” volcanism are located near Mars’s equator, not its poles.
Holt introduced multiple different objections to the liquid water hypothesis, starting with the lack of geological evidence. Subglacial lakes on Earth are overlain by layers that have “drawn down” over time as layers above the lake undergo melting, but there’s no evidence of this drawing down on Mars. Similarly, there are no springs present due to flowing water. Holt pointed out that there are many bright radar echoes in the south polar layered deposits, some of which are located close to the edge where the ground is less insulated and temperatures are far too cold for even fully saturated brines to be liquid. Past research has also suggested that if the entirety of Mars were covered with layered deposits as seen at the south pole, much of the planet would have similarly bright radar echoes without the need for liquid.
Where do we go from here? Both teams mentioned the Shallow Radar, or SHARAD, instrument on the Mars Reconnaissance Orbiter, which operates at a higher frequency than the Mars Advanced Radar for Subsurface and Ionosphere Sounding (MARSIS) instrument whose data were scrutinized in this session. If SHARAD can detect echoes from beneath the south polar cap, the frequency-dependent behavior of the material should help us unravel the mystery of its composition. So far, no high signal-to-noise echoes have been reported, though experimenting with a new technique in which the spacecraft rolls by 120 degrees has been promising. With more than 20 research articles already published on this topic, we can expect to hear many more arguments in the case of Mars’s south polar water!