Editor’s Note: Astrobites is a graduate-student-run organization that digests astrophysical literature for undergraduate students. As part of the partnership between the AAS and astrobites, we occasionally repost astrobites content here at AAS Nova. We hope you enjoy this post from astrobites; the original can be viewed at astrobites.org.
Title: Analysis of a JWST NIRSpec Lab Time Series: Characterizing Systematics, Recovering Exoplanet Transit Spectroscopy, and Constraining a Noise Floor
Authors: Zafar Rustamkulov et al.
First Author’s Institution: Johns Hopkins University
Status: Published in ApJL
JWST is the most powerful infrared telescope ever built — and, understandably, scientists across the world and in every sub-field of astronomy can barely contain themselves waiting for the data it will soon beam back. The gorgeous shots taken during the commissioning phase for engineering and alignment suggest the measurements will be exquisite. But how exquisite, exactly? What are the subtlest signals JWST will be able to detect, specifically in the context of exoplanet atmospheres? Today’s authors take a step towards answering that question — and, impressively they do it before any scientific measurements have been taken!
How Good Is Great?
As capable as JWST is, you could probably guess that it can’t do everything we could possibly ask of it. Although the telescope can take images of the most distant galaxies across the largest scales of the universe, all of its measurements come with some amount of uncertainty, or noise. If that noise is small compared to the signal we’re trying to measure, then we don’t need to worry; for example, if we measure the age of a rock to be 5 million years ± 0.01 million years, we can be confident that it’s a young rock even if we aren’t exactly sure of its age. But, if we measure it instead to be 2 billion years ± 2 billion years, suddenly our measurement looks less useful, since the rock could either be very young or nearly as old as Earth itself!
In the context of JWST and astronomy, if you measure, say, 100 photons per second from a galaxy or star, what are the chances that source is actually shooting 100 photons per second in our direction? Could it be 100 ± 50 photons per second? If you’re trying to measure a very subtle signal (say, the transit of a planet) that would only block say 40 photons per second, the answers to those questions could make the difference between confidently detecting your planet and not being sure if it was even there! So, what are the answers? The only way to know is to analyze actual measurements taken by JWST. You would think that means we have to wait until the first science images arrive this summer. Unless…
Blinded by the (Lamp) Light
Although JWST is now in its chilly home out at L2, it somewhat infamously took many years to reach this point. One reason for this was the extensive testing of every component of the telescope. As part of this testing regimen, engineers locked the science instruments in a chamber meant to replicate the cold, vacuum-like conditions of space, then ran them through their paces to check their response. While the science instruments were in the chamber, the engineers forced the Near Infrared Spectrograph (NIRSpec) instrument to undergo the worst eye exam ever, shining a tiny lamp through its optics and onto its detector for several hours.
But ah ha! What if we just pretended that chamber actually was space, and that little lamp was actually a star? If we could clean up this data, add in a fake but perfectly known transit signal, and then check how well we could recover that true signal, we’d have an estimate of NIRSpec’s noise resolution. This is exactly what today’s authors set out to do.
This wasn’t straightforward, since lamps, unfortunately, are not stars. NIRSpec breaks light into its component wavelengths to measure its spectrum, and a glowing filament produces a very different spectrum than a star. Even worse, lamps flicker and change over time, but contrary to what popular children’s songs would suggest, stars don’t actually twinkle in space. The authors took great care to remove each of the effects that could influence the results, sliding and smoothing each of the frames until they resembled something similar to what we’ll see from a real star in space. Figure 1 shows the brightness of the lamp after the authors applied various corrections.
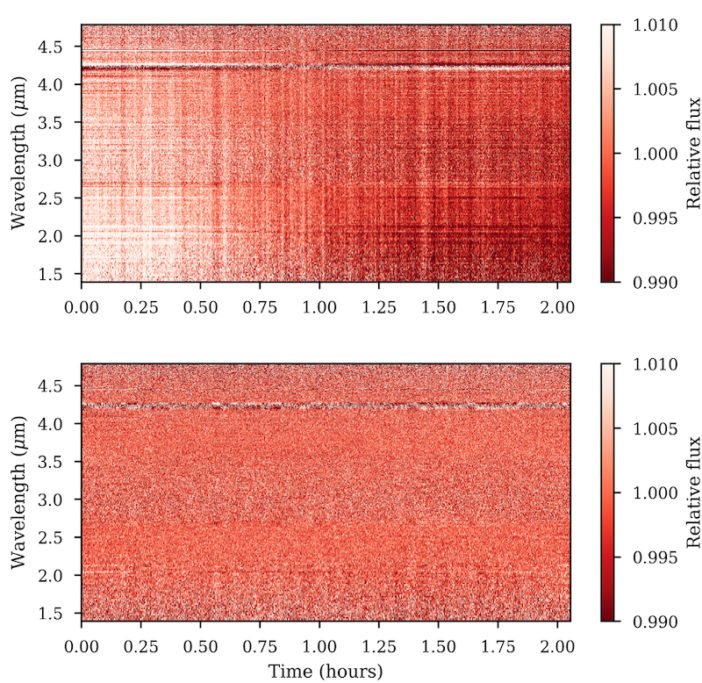
Figure 1: The lamp data before (top) and after (bottom) trend removal. In both panels, each column of pixels represents one integration, then they are lined up left to right in the order they were taken. Note that in the top panel, representing data before a trend removal step, the average column intensity goes down over time due to the lamp fading slightly. In the bottom panel, showing data after their “common-mode correction,” which “mostly removes the systematics imparted by the unstable light source,” the source appears much more stable. [Adapted from Rustamkulov et al. 2022]
Noise Canceling Telescope
So, what did the authors find? Lots of very good news! Despite the lamp drifting around the image plane more than we expect stars will, the authors found that NIRSpec should be able to detect spectral features from the atmospheres of TRAPPIST-1 d and GJ 436 b, as shown in Figure 2.
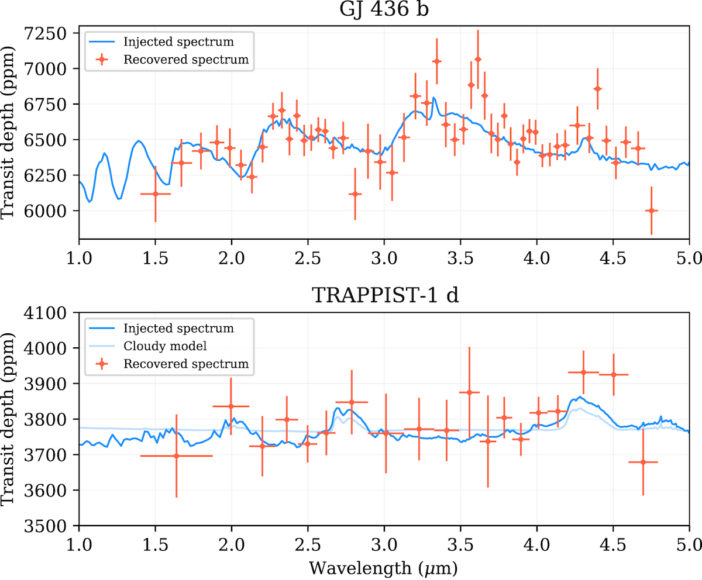
Figure 2: The recovered spectra of two planets. In both panels, the blue curve depicts the “true” signal that the authors injected into their lamp data, and the red points are the results of their model fit to those fake measurements. The Y axis here is transit depth, or how much of the star’s light at a given wavelength is blocked by the planet, in parts per million (ppm). Note how closely the recovered points follow the true curves, especially in the case of GJ 436 b between 1.5 and 3 microns. [Rustamkulov et al. 2022]
The excitement and anticipation for spectra of exoplanet atmospheres seems justified! Back to waiting for the first science images…
Original astrobite edited by Jessie Thwaites.
About the author, Ben Cassese:
I am a first-year Astronomy PhD student at Columbia University working on simulated observations of exomoons. Prior to joining the Cool Worlds Lab, I studied Planetary Science and History at Caltech, and before that I grew up in Rhode Island. In my free time I enjoy backpacking, spending too much effort on making coffee, and daydreaming about adopting a dog in my NYC apartment.