Editor’s note: Astrobites is a graduate-student-run organization that digests astrophysical literature for undergraduate students. As part of the partnership between the AAS and astrobites, we occasionally repost astrobites content here at AAS Nova. We hope you enjoy this post from astrobites; the original can be viewed at astrobites.org.
Title: A Revised Description of the Cosmic Ray-Induced Desorption of Interstellar Ices
Authors: Olli Sipilä, Kedron Silsbee, Paola Caselli
First Author’s Institution: Max Planck Institute for Extraterrestrial Physics, Germany
Status: Accepted to ApJ
Cosmic Ray Desorption
To first understand the origins of life, we must first understand the origins of life-sustaining molecules. Complex Organic Molecules (COMs) are a common group of carbon-based species that are often considered to be the first steps of sustaining molecules. COMs have been observed in the gas phase in space, but chemical models indicate that COMs have to form in the ice layers of dust grains, rather than in the gas. A common question astronomers seek to answer is: How do molecules, like COMs, go from the ice phase to the gas phase?
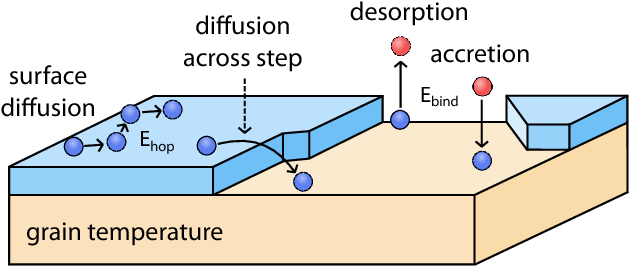
Figure 1: On grain surfaces, molecules and atoms can move around (diffuse), react, stick to the surface (accrete), and desorb into the gas phase. [van Dishoeck 2014]
This process, known as desorption (Figure 1), can occur several different ways, but for today’s bite we’ll focus on cosmic ray desorption. Cosmic rays are high energy particles (mostly hydrogen and helium) that can strike dust grains and deposit energy. The deposited energy is then converted to thermal energy, thus heating the grain. Dust grains will then shed the heat to “kick off” molecules (like COMs) from the ice into the gas phase. The rate at which desorption occurs can be directly measured by the grain cooling time, which is determined by the composition of the ice on the grain. If the ice is made of species that strongly bind to the grain (known as a high binding energy), then it takes longer for the grain to cool. Oppositely, if the ice is made of species that loosely bind to the grain (have low binding energies), then cooling and desorption occur more quickly.
Modeling Grain Heating
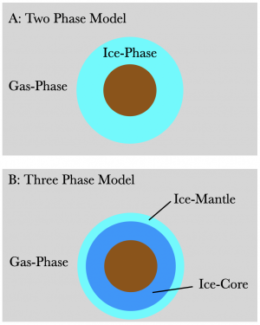
Figure 2: (a): Two-phase chemical model. For cosmic ray calculations, the ice is typically assumed to be CO. (b) Three-phase chemical model consisting of two layers of ice, where mixing between the mantle and core can occur. This allows for a diverse (and more realistic) ice mixture. [Astrobites]
Today’s paper challenges the assumption that ice layers are dominantly CO and introduces a dynamic cool-down rate that is dependent on the ice composition. The authors seek to understand whether a more realistic representation of ice could affect the rate at which molecules desorb to the gas phase, or whether the previous assumptions instead hold.
To test this, today’s authors ran 4 different models, including a two-phase and three-phase fiducial (Figure 2b) model based on Hasegawa & Herbst (1993) and a two-phase and three-phase dynamic model, with a variable grain cooling rate. The ice layers used in these models included species commonly known to exist in the ice layers on dust grains, not just CO. For example, their model included carbon, nitrogen, and oxygen, as well as ammonia, methane, and water, among other species.
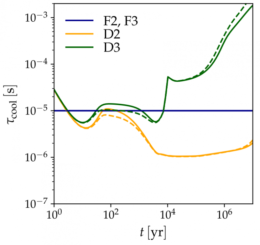
Figure 3: This plot shows the different cool-down rates (y-axis) for the 4 models over time (x-axis). Since the dynamic models have variable cool-down rates, the cool-down rates change over time. Note that the fiducial models (F2 and F3) have constant cooling rates, the two-phase dynamic model is faster (D2), and the three-phase dynamic model is slower (D3). [Sipilä et al. 2021]
Cooling Down
As expected, the fiducial models yielded a constant cool-down rate of 10-5 sec. However, the two-phase dynamic model had faster cooling rates, while the three-phase dynamic model had slower cooling rates (Figure 3). The different cooling rates are reflected in the desorption rates of different molecules, where the three-phase fiducial model typically had the slowest desorption rates (Figure 4).
The dynamic models yielded different results from the fiducial models because molecules and atoms frozen out are not “stuck” in place in the dynamic models. In reality, species frozen on grains can migrate, which causes a dynamic and variable set of binding energies to be used in the grain cooling rate calculation. Because of this, the dynamic models produce variable cooling rates compared to the fiducial model, which assumes that the ice is stagnant.
The two-phase fiducial model yields faster cooling times, likely because species with low binding energies, like CO, dominate the ice layer. The three-phase fiducial model, with two layers of ice, allows for species with higher binding energies, like ammonia, to slow down the grain cooling rate.
While we observe a diverse number of chemical species in the gas phase, the majority of these species, such as COMs, form in the ice layers of dust grains. To best understand the past of life-sustaining molecules, we must first understand how complex and diverse ices are desorbed into the gas. Today’s paper introduces a new, easy to apply dynamic cosmic ray desorption that is likely more accurate to real astronomical conditions.
Original astrobite edited by Jason Hinkle.
About the author, Abygail Waggoner:
I am a second year chemistry graduate student at the University of Virginia and NSF graduate fellow. I study time variable chemistry in protoplanetary disks. When I’m not nerding out about space, I’m nerding out about fantasy by reading or playing games like dungeons and dragons.