Since beginning operation, gravitational-wave observatories have observed several mergers involving neutron stars and black holes. Both black holes and neutron stars are the result of supernovae, so is it possible for us to identify a pair of these objects pre-merger?
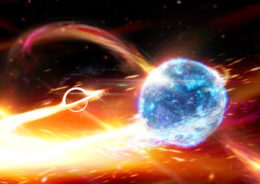
An artist’s impression of a black hole–neutron star binary. [Carl Knox, Arc Centre Of Excellence For Gravitational Wave Discovery (Ozgrav) At Swinburne University Of Technology]
Some Go Supernova First
The first black hole–black hole (BH–BH) merger was detected by the Laser Interferometer Gravitational-wave Observatory (LIGO) in 2015. Since then, LIGO and the Virgo interferometer have observed several BH–BH and neutron star–neutron star (NS–NS) mergers. Interestingly, the two observatories have also found candidates for BH–NS mergers. So how are the progenitors of these mergers formed?
One possibility is that black holes and neutron stars encounter each other in densely populated areas of space and just happen to pair off. Another possibility is that these pairs of dense objects start off as massive stars in a binary and evolve until they reach their pre-merger form.
Both scenarios involve supernovae, as the stars evolve to become neutron stars or black holes. But there’s an interesting consideration for the latter scenario, if one star becomes a black hole before the other finishes evolving: How would the black hole interact with the supernova caused by its companion?
In a recent study, a group of researchers led by He Gao (Beijing Normal University, China) tackle that question.
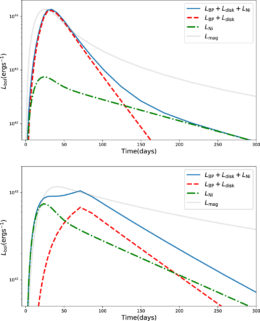
The light curves from two instances of a black hole interacting with material ejected from its companion’s supernova. In the upper panel, the energy from the interaction is larger than the energy associated solely with the supernova. In the lower panel, the energy from the interaction is comparable to the energy associated with the supernova. LBP is the brightness from black hole outflows, Ldisk is from the black hole’s accretion disk, and LNi comes from the radioactive decay of nickel associated with the supernova. Lmag is the total brightness from the system. [Gao et al. 2020]
Just Add Ejected Mass
Gao and collaborators first estimated how much mass and energy would be released by a massive star going supernova. They also put constraints on the velocity of the ejected mass, since it would play an important role in determining any interaction with the black hole.
If any material fell into the black hole’s sphere of influence, it would result in energy being released in multiple ways, like through jets and outflows. Gao and collaborators determined that these releases of energy could happen on timescales similar to the supernova. So what do you get when you look at the total energy released by the merger progenitor?
Energetically Interfering with Supernovae
If we plot the brightness of a supernova from start to finish, we get a light curve that peaks very quickly and then slowly tapers off. This curve can change based on the type of supernova involved, but broadly speaking, most supernovae have this characteristic shape in brightness–time space.
In the merger progenitor, energy released by ejected material interacting with the black hole would disrupt this characteristic supernova light curve. The extent of this disruption would depend on a variety of factors, but Gao and collaborators noted that at least a small fraction of these disrupted supernovae could be detected.
If we observed a number of these disrupted supernovae, we could compare the rate at which they occur to the rate of relevant mergers being detected by gravitational-wave observatories. The result could point us towards one of the two scenarios that produce merger progenitors. So, as with most things astronomy, more observations please!
Citation:
“Special Supernova Signature from BH–NS/BH Progenitor Systems,” He Gao et al 2020 ApJL 902 L37. doi:10.3847/2041-8213/abbef7
4 Comments
Pingback: From AAS NOVA: “The Signature of a Pre-Merger System” | sciencesprings
Pingback: Czy możliwe jest wykrycie pary czarna dziura-gwiazda neutronowa przed ich połączeniem? – PTMA Kraków
Pingback: Astronomia Śląska
Pingback: Czy możliwe jest wykrycie pary czarna dziura-gwiazda neutronowa przed ich połączeniem? – Astronomia Śląska