Editor’s Note: This week we’re at the 236th AAS Meeting, being conducted virtually for the first time! Along with a team of authors from Astrobites, we will be writing updates on selected events at the meeting and posting each day. Follow along here or at astrobites.com. The usual posting schedule for AAS Nova will resume the week of June 8th.
Welcome Address (by Sanjana Curtis)
AAS President Megan Donahue (Michigan State University) kicked off the 236th meeting of the American Astronomical Society — the first AAS meeting that is entirely virtual. Before opening the meeting, she acknowledged the fact that many members of the astronomy community are not present due to a variety of factors, including a pandemic, the choices of others, and an uninterrogated system that grants privileges to a select few. This was a sobering reminder that science is, above all, a human endeavor.
As a virtual meeting, this AAS has a low carbon footprint that is certainly good news for the environment. At the same time, the meeting attendance is almost double the usual attendance at in-person meetings, including twice as many students! There are scientists from 34 countries and 49 of the 50 US states (with the exception of Alaska) in attendance. The schedule is packed, the science is exciting, and we’re looking forward to 3 days of hearing about the latest research and the newest results in astronomy!
Fred Kavli Plenary Lecture: Searching for Habitable Worlds: Challenges, Opportunities & Adventures (by Sanjana Curtis)
Lisa Kaltenegger (Carl Sagan Institute) delivered the Fred Kavli Plenary Lecture and spoke about the challenges, opportunities, and adventures to be found in our search for habitable worlds. Starting from being able to observe just a handful of exoplanets, we have now reached a point where we can start doing statistics with them. We have found thousands of new worlds that show great diversity in their properties, and exploring this diversity through theoretical modeling can help us understand the physics of both the planets and their host stars. A wonderful review on this topic can be found here.
Dr. Kaltenegger also addressed whether we can distinguish the population of rocky planets from the population of gas planets based on the mass and radius of the exoplanet. If we know both the mass and the radius of an exoplanet, we can derive its mean density and composition, and compare it to planets in our solar system. However, for most exoplanets, either the mass or the radius is known depending on the detection method. In general, the line dividing gas planets from rocky planets sits at around 2 Earth radii—all exoplanets below this limit are rocky planets.
The spectra of exoplanets are key to understanding their properties and Dr. Kaltenegger’s research group is creating a database of spectral fingerprints to compare against future observations by telescopes like ELT or JWST. These data are freely available here. She stressed that we do not just have the current Earth as a template for habitable planets, but also the Earth through time.
She ended her talk with some intriguing and adventurous ideas: the possibility that organisms on planets around active M stars may develop biofluorescence to protect themselves from harsh ultraviolet light, and the existence of habitable worlds around red giants and white dwarfs!
Press Conference: Galaxies Weird & Wonderful (by Haley Wahl)
The second press conference of the day involved new studies on some galactic oddities! Four scientists took turns sharing what they’ve discovered lurking in our cosmic neighborhood.
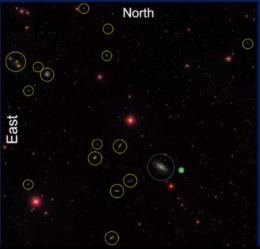
Satellite galaxies of NGC2998 have a “lopsided” distribution. This image shows how there seem to be more satellite galaxies (circled in yellow) on one side of the host galaxy (circled in blue) than the other.
First, Tereasa Brainerd (Boston University) shared her team’s findings that involved the distribution of satellite galaxies around a host galaxy. Scientists believe that the dark matter halos that surround galaxies (as predicted by the cold dark matter theory) are spherical, flattened elliptical halos. By looking at the systems with 5+ satellites around the host galaxy, they found “lopsided” distributions, meaning there were more pairs of galaxies on one side of the galaxy than the other. Could this have implications for cold dark matter? It’s possible!
Next up was Lior Shamir (Kansas State University) who continued the theme of asymmetries. He presented observations that showed that the distribution of apparent spin directions of galaxies isn’t always symmetric, that it changes depending on the direction of observation. He also showed that the direction of spin is linked to its brightness and that asymmetry varies with redshift. Press release
The third speaker was Sanskriti Das (Ohio State University), who talked about evidence for a massive hot circumgalactic medium around a luminous star-forming galaxy. She and her team showed, using X-ray data, that this material, which is extended over a large volume and has a large mass (comparable to the mass of all the stars in that galaxy!), could be the solution to the “missing baryons” problem in galactic halos. Press release
The last speaker of the press conference was Ripon Saha (University of Missouri, Kansas City), who presented the discovery of a massive proto-supercluster 10 billion light-years away. Using a pipeline made using dust-obscured galaxies (DOGs), he and his team identified the proto-supercluster of galaxies surrounded by nascent ones. This is the first time any observed cluster has been found embedded in its birth environment, which could give astronomers a unique opportunity to study such a cluster.
Plenary Lecture: The Inclusion Revolution (by Luna Zagorac)
The midday plenary entitled “The Inclusion Revolution” was delivered by Dr. Dara Norman, the Deputy Director of the Community Science and Data Center at NSF’s NOIRLab in Tucson, AZ. Dr. Norman began her plenary by describing the term “culture”: the customs, institutions, and achievements of a group of people. As scientists and astronomers, we have our own culture — including our language, our traditions, our norms, our values, and even our art — and parts of this culture must be changed to support diversity and inclusion in astronomy. Indeed, culture is not a static object: an example of cultural change comes from the very first decadal survey, which addressed the demographics of the workforce and the lack of availability of public telescopes. This led to the funding of the national observatories, without which the state of astronomy today would look completely different, underscoring the potential impact of the 2020 and future decadal surveys.
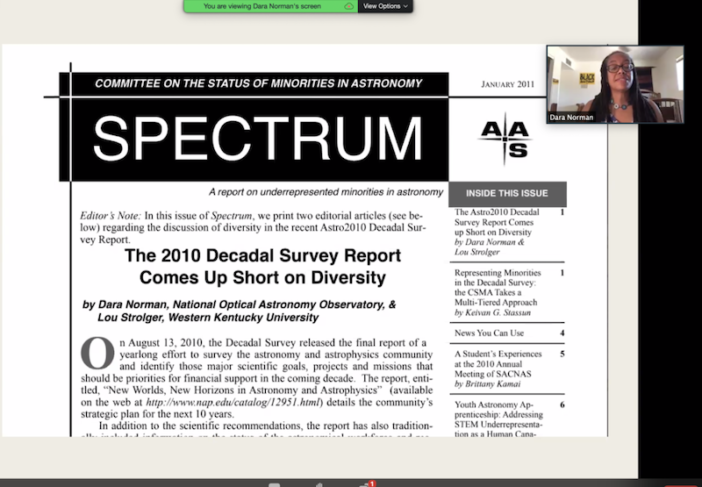
The AAS decadal survey has always been a tool for assessing the demographics of astronomers conducting research, and for changing culture. Indeed, the first one ever published fueled the founding of national observatories due to lack of access to telescopes nationwide!
A point that Dr. Norman emphasized throughout her plenary is a quote from Paula Stone Williams’s TED talk — “I didn’t know what I didn’t know.” The first step towards inclusion is thinking about not just who is represented on policy-making committees, but what kind of expertise is represented. It’s not enough to have a lot of different voices if there isn’t a critical mass of expertise in the room. Additionally, we need to empower each representative to act as a mouthpiece for their community, while gathering ideas and thoughts from the community. In this way, the issue of having too many people on committees can be avoided, and the workload redistributed amongst individuals.
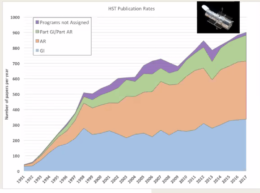
The blue area represents the papers written on data from dedicated HST observing runs, while the orange represents papers from archival data. Note that the numbers equal out with time!
Next, Dr. Norman discussed her paper (Norman 2018), which explored how to use big data to reach insider status in the field — including being invited to join collaborations, give talks, review papers, and more. She found that, with time, the number of papers published on data from allocated Hubble time evened out with the number of papers written from archival data. Findings from Peek et al. (2019) broke that down by authorship, and the gap was significantly larger at small and underfunded institutions. This raised the question of whether archival data can be used as a vehicle to accessing the dedicated observation time that is critical for advancing in the field.
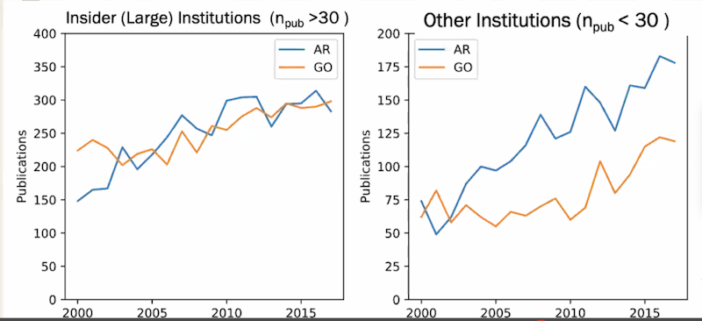
Figure from Peek et al. 2019. The blue line represents HST papers published using archival data, while the orange represents papers stemming from dedicated observing time. Note the discrepancy between large and small institutions!
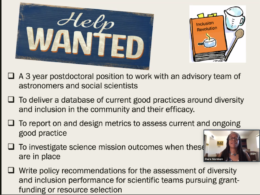
Dr. Norman is looking for a 3-year postdoc (click to enlarge)! The posting is not out yet, but you can contact Dr. Norman via email for more details.
Going back to the general theme of “you don’t know what you don’t know,” the first step to bridging this gap is to directly ask colleagues at small and underserved institutions what they need. One of the needs Dr. Norman identified was the need for more policies and incentives for R1 institutions to work with smaller institutions, as is currently rarely the case. To this effect, Dr. Norman will be working on a toolkit for effective diversity and inclusion practices and their efficacy, particularly aimed at underserved institutions. She will be looking for a 3-year postdoc to aid in this effort, and can provide more information upon email request.
Dr. Norman ended her talk by underscoring that there is much more work to do on diversity and inclusion in astronomy, including through the decadal surveys, which have been a tool for demographics-driven cultural change from the very start. She closed by reminding us that we need change; that the revolution will not simply be tweeted; that black lives matter; and to vote.
NASA Town Hall (by Abby Waggoner)
The NASA Town Hall was led by Paul Hertz, Director of the Astrophysics Division in the Science Mission Directorate at NASA. The NASA Town Hall provided an overview of NASA operations, funding, and missions from the past year, along with goals and funding for the future. Below, each topic covered is summarized in bullets. All images are obtained from the slides provided during the town hall.
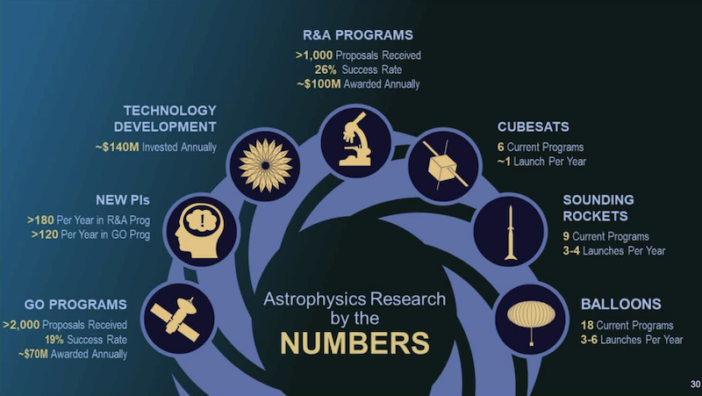
Where does NASA Astrophysics funding go? The numbers shown in this figure shows that the funding goes to supporting many scientists and projects!
Committed to Improving
- Mission PI Development: NASA seeks to increase the diversity of mission principal investigators.
- Fellowships and funding for graduate students and postdoctorates were awarded, including:
- Nancy Grace Roman Technology Fellowship
- Hubble Fellowship
- NASA Earth and Space Science Fellowship (NESSF)
- Future Investigators in NASA Earth and Space Science Technology (FINESST).
- Peer reviews have become anonymous.
Mission Program Update
- Impact of COVID-19: Flight operations have continued nominally with the exception of the Stratospheric Observatory for Infrared Astronomy (SOFIA has been grounded; see below).
- Priority has been given to Mars 2020 and the James Webb Space Telescope (JWST).
- Updates from missions currently in flight:
- Hubble had its 30th birthday. You can find out what Hubble was imaging on your birthday here.
- The Transiting Exoplanet Survey Satellite (TESS) has discovered 47 confirmed planets and 1,837 planet candidates. The four-planet system HD 108236 was highlighted, as the three outer planets are candidates for spectral analysis with JWST.
- SOFIA has suspended flight operations since March 2020 due to COVID-19. There will be a community update on SOFIA on June 2 at 3pm (EDT) during AAS 236.
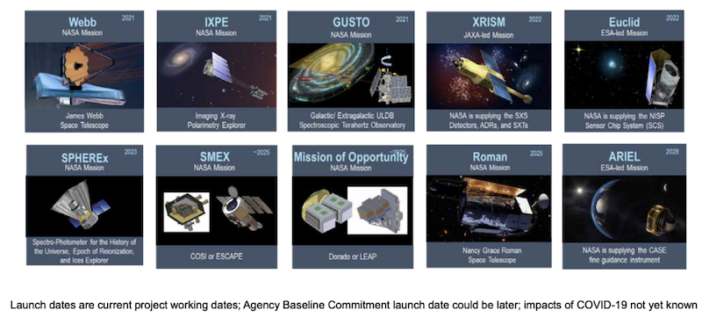
NASA has 10 missions planned for the future. These missions include a science goals ranging across the entire electromagnetic spectrum.
- Updates from among NASA’s 10 missions currently in development:
- JWST has undergone deployment testing, and will soon begin observatory-level environmental testing.
- Roman Space Telescope (formerly Wide-Field IR Survey Telescope, or WFIRST) is fully funded and in production. Roman will aid in understanding the evolution of the universe to exoplanet direct imaging.
- Two small explorers are planned:
- ESCAPE will investigate UV stellar flares and their impact on the habitable zone.
- COSI will observe MeV gamma-rays to trace the Milky Way’s supernova activity.
- Two missions of opportunity are planned:
- Dorado will watch for UV light emitted by merging neutron stars.
- LEAP will be attached to the ISS and observe the polarization of gamma-ray bursts in jets.
Planning for the Future
- NASA funding for the Astrophysics Division is at an all time high, with 58% of the funding dedicated to JWST and Roman.
- NASA seeks to have humans sent to the moon by 2024 through the Artemis Project.
- In the next decade, NASA has concepts for several medium and large missions in the 2020 Decadal Survey.
Press Conference: Cosmic Bangs & Whimpers (by Alex Pizzuto)
Deborah Schmidt (Swarthmore College) kicked off this afternoon’s press conference by summarizing her dissertation work, which focuses on the abundance of different atomic isotopes in planetary nebulae. While many of us may know of planetary nebulae, such as the butterfly nebula, for their strikingly beautiful silhouettes, these objects “also play a significant role in the recycling of matter in our universe,” according to Schmidt.
Planetary nebulae are the final stages of Sun-like stars. Towards the end of their lives, stars can eject material that flows away from the remnant core and enriches nearby interstellar regions, filling it with a wide array of elemental isotopes that were originally produced in nuclear reactions within the star. Until recently, it was thought that these isotopes shouldn’t linger very long, because the hot white dwarf remnant at the center of this nebula should produce a plethora of high-energy radiation, which has the potential to destroy the expelled molecules.
Schmidt presented recent radio observations that challenge these beliefs, and found that these regions, although expected to be cleared out of these elemental abundances, were instead “bursting with molecules.” This wealth of elemental isotopes was found in both the young and old nebulae that Schmidt studied, and this discovery has major implications for a breadth of topics. These include potentially remedying tensions relating to the prevalence of poly-atomic ions in the diffuse interstellar medium, as well as explaining the previously anomalous origins of dust grains that are present even in our own solar system. Additionally, these observations allowed Schmidt and collaborators to build a new model of the inner workings of planetary nebulae, and to show how potentially explosive origins can not only explain the prevalence of a variety of elemental isotopes, but can also explain the gorgeous shapes of planetary nebulae that we know and love.
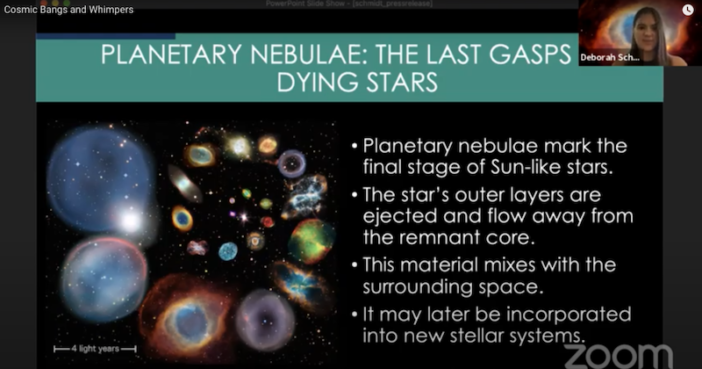
Schmidt describes the plethora of elemental isotopes that originate in planetary nebulae and explains how these objects explain the anomalous abundances of elements in the diffuse interstellar medium.
Adelle Goodwin (Monash University) then went on to describe recent observations of extremely energetic outbursts visible across nearly the entire electromagnetic spectrum: accreting pulsars. Accreting pulsars consist of neutron stars, the dense remnants of dead stars, in close orbit with a “normal” star. Neutron stars are so dense that a mere handful of their material would have the same mass as about 5 Mount Everests, or 5 billion tons. If in orbit with a nearby star, these neutron stars can slowly consume their companions, filling up accretion disks until reaching a critical threshold, and then quickly gobbling up some of this material and expelling a fraction of it in a violent outburst.
This outburst should be visible in a variety of electromagnetic wavelengths, including optical, UV, and X-ray, and the evolution of the radiation in these different wavebands reveals a wealth of information about the underlying processes that are occurring. Goodwin describes the first ever set of observations of this entire outburst period with simultaneous coverage in these various wavelengths. Specifically, her team targeted a pulsar located 11,000 light-years away, spinning 400 times per second, and they observed this pulsar with more than 7 telescopes. As Goodwin and her team investigated this object, they noticed that the X-ray emission began at a time much later than the optical emission, with a delay of about 12 days, longer than any model would have predicted. The team was able to reconcile this discrepancy by suggesting that the disk consists of a large fraction of helium, which takes longer to heat up and then ionize. Regardless of the delay of X-rays, these observations of an outburst thousands of times brighter than the Sun marks a new step in understanding the dynamics of these explosive transients. Press releaseNext up was Justin Vandenbroucke (University of Wisconsin, Madison) on behalf of the Cherenkov Telescope Array (CTA), a team of scientists dedicated to investigating the universe at the highest energies. Some objects in our own galaxy are capable of producing particles of light with energies exceeding one tera-electronvolt (TeV), which is about one trillion times more energetic than the particles of light we detect with our own eyes. Studying this light, and the objects capable of producing it, teaches us about the most efficient and energetic accelerators in our universe.
In order to detect these particles, Vandenbroucke described a prototype ground-based gamma-ray telescope. When a gamma ray approaches the Earth, it can demolish an atom in the atmosphere. This collision produces a menagerie of particles travelling at nearly the speed of light, which in turn produce tiny flashes of blue light. The goal is to then reflect these tiny blips of blue light into a camera by building precise and gargantuan mirrors on the ground. In case that does not sound difficult enough, Vandenbroucke described how this telescope features a two-mirror design as well as state-of-the-art electronics. This symphony of advanced electronic and complicated optics should be able to come together to produce some of the most vivid and detailed pictures of the universe in its most energetic states.
To test out this new telescope, Vandenbroucke and his team pointed their telescope at the Crab nebula, the remnant of an exploded star from 1,000 years ago, which features a pulsar in the center powering very bright emission up to TeV energies. The team was able to significantly detect this object at these amazingly high energies. Vandenbroucke noted that this “detection establishes innovative telescope technology for gamma-ray astronomy.” Together with the other telescopes being constructed, the CTA consortium should revolutionize the way we see the universe and will play a pivotal role in both multi-wavelength and multi-messenger astronomy at the highest energies. Press release
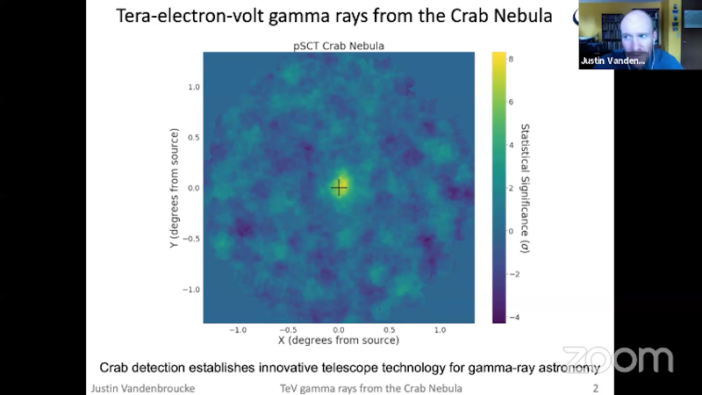
Vandenbroucke shows the first detection of the Crab nebula with this revolutionary high-energy gamma-ray telescope, providing a picture of this object at energies trillions of times more energetic than we detect with our eyes.
Wrapping up today’s press conference was Fabio Pacucci (Black Hole Initiative & Center for Astrophysics), discussing how black holes grow. We know that black holes come in a variety of masses, ranging from those just a few times more massive than the Sun all the way to millions of times more massive than this. What we don’t know is how these black holes get to be so large.
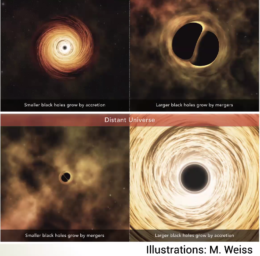
Fabio Pacucci’s conclusions for how black holes of different sizes grow at different times in the universe. Click to enlarge and read text. [Illustrations: M. Weiss]
Pacucci found that for nearby black holes, lighter black holes are more likely to gain mass via accretion and heavy black holes via mergers. What’s bizarre is that if you peer out to black holes farther away from us, then the exact opposite is true, namely distant, light black holes are likely to acquire mass from merging and distant, heavy black holes are likely powered by accretion. This is important because the growth mechanism has implications for the spin of these systems, as black holes that grow from accretion are likely to spin much faster than those that grow from mergers. This has direct observational consequences, as spinning black holes should be more efficient at emitting radiation and thus may be easier to observe with electromagnetic observatories, whereas black holes that are growing from mergers are likely best detected with gravitational wave detectors. Pacucci summarized this nicely, noting that “this [study]will inform decisions regarding observational strategies with future space telescopes, as well as lay the basis for models that describe other aspects of the evolution of the universe.” Press release
Plenary Lecture: Journey to the Center of the Galaxy: Following the Gas to Understand the Past and Future Activity of Galaxy Nuclei (by Abby Waggoner)
Our final plenary lecture of the day was given by Elisabeth Mills from the University of Kansas. Dr. Mills discussed the “Journey to the Center of the Galaxy: Following the Gas to Understand the Past and Future Activity of Galaxy Nuclei” and brought us from our solar system to the center of the galaxy, 26,000 light-years away. In this journey, we explored how different wavelengths of light can be used to understand the past (and future) of a black hole in the center of a galaxy.
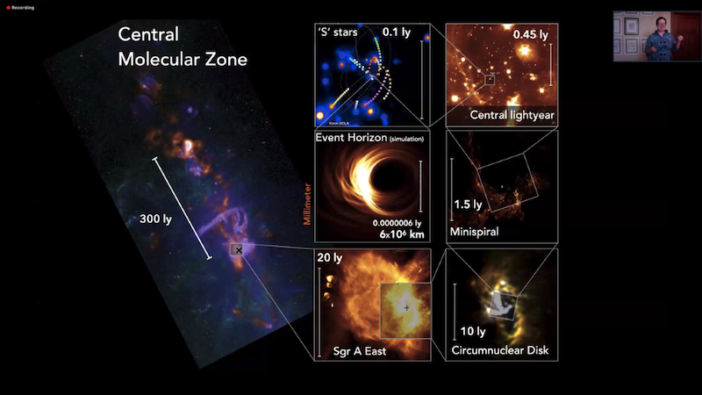
The center of our galaxy, zoomed further and further in at different wavelengths. Note that we use different types of light for each image, to see further and further into the center.
When we look towards the Milky Way’s black hole, Sgr A*, clouds of dust between us and the black hole absorb all optical light, so we have to use other wavelengths of light — such as infrared, radio, and millimeter — to observe the galactic center. Each type of light tells us something different about what’s happening in the center of the galaxy. Radio wavelengths show us the light of past generations of stars, or those that have died and experienced a supernova. Infrared wavelengths show us the “stars of today,” by letting us see the dust warmed by stellar radiation. Millimeter wavelengths show us the “stars that will be” by revealing the location of cold gas and dust that will eventually collapse to form a star. Together, these wavelengths will help us in understanding the past, present, and future activity of the black hole in the center of our galaxy.
The gas and dust in the center of our galaxy is hotter, denser, and more turbulent than the gas and dust in the interstellar medium. This raises the question: Why is the dust at the center of the galaxy so different?
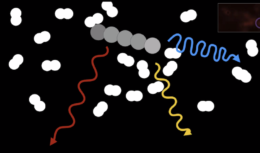
As H2 molecules collide with HC3N, HC3N rotates and emits energy. More dense regions result in more collisions, and the emission of light with a lower wavelength.
To answer this, astronomers consider the light emitted by rotating molecules, such as HC3N. When molecules collide with other molecules (such as H2), the kinetic energy of the collision causes the molecule to spin, or become “rotationally excited.” When there are a lot of collisions, such as in a high density region, the molecule spins at a higher frequency/energy. This allows us to probe the density of dust and gas near the center of the galaxy by observing these different rotation energies.
When astronomers look towards the center of the galaxy, we find that the gas and dust density drastically drops around 350 light-years from Sgr A*, where more than 99.9% of all the dust mass in the center of the galaxy is beyond 350 light-years from the black hole. This is evidence of strong intense shocks released by Sgr A*. However, our black hole is currently not active enough to explain this mass drop. This indicates that our black hole wasn’t always “boring,” and used to be much more active.
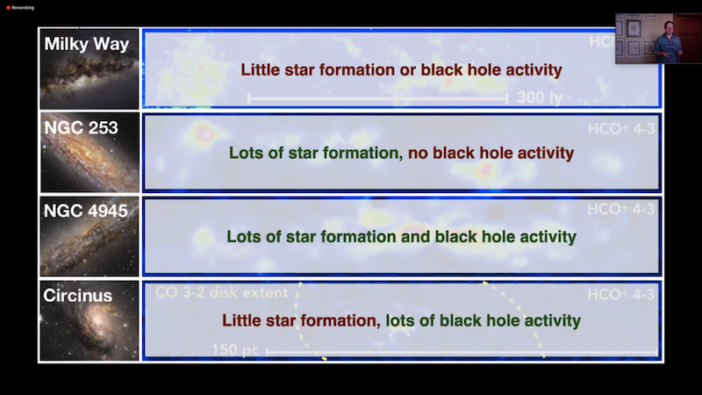
Based on observations of these four galaxies, we can see a variety of black hole activity and star formation. This allows us to get a more clear picture of the evolution of galactic centers.
Thanks to telescopes such as ALMA and Hubble, this technique can also be used to explore black holes in other galaxies, thus giving us a broader picture and understanding of the evolution of galaxies.
2 Comments
Pingback: Allgemeines Live-Blog ab dem 2. Juni 2020 | Skyweek Zwei Punkt Null
Pingback: So „war es“ auf der ersten virtuellen AAS-Tagung | Skyweek Zwei Punkt Null