Editor’s Note: This week we’re at the 234th AAS Meeting in St. Louis, MO. Astrobites and AAS Nova will be working together to publish updates on selected events at the meeting this week; the usual posting schedule for AAS Nova will resume next week.
Laboratory Astrophysics Division (LAD) Plenary Lecture: The Role of Dust in the Molecular Universe (by Kerry Hensley)
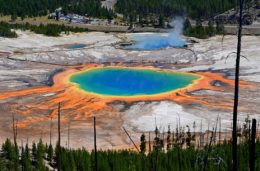
Grand Prismatic Spring in Yellowstone National Park. [Clément Bardot]
These discoveries give us a hint — and hope — that life may be widespread in the universe, able to withstand even the harshest environments. Life on Earth is built from organic molecules, which are composed of carbon, hydrogen, nitrogen, oxygen, phosphorous, and sulfur. How did the organic molecules that life is built from end up on Earth in the first place? They can either exist in the material that the Earth formed from (starting with tiny dust grains in the Sun’s protoplanetary disk) or they can be delivered to the planet later on in the form of asteroids and comets (particularly the ones that were scattered into the inner solar system during the Late Heavy Bombardment).
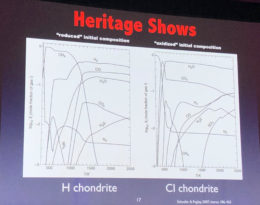
An example of how the composition of chondrites (stony meteorites) can affect the composition of a planet’s
atmosphere.
The chemical makeup of this material is important because it determines, in part, the composition of Earth’s atmosphere. For example, if most of the material has a “reduced” composition, the atmosphere will tend to be methane-rich. If most of the material has an “oxidized” composition, the atmosphere will tend to be nitrogen-, carbon-dioxide-, or water-vapor-rich, depending on the temperature. It’s really easy to make amino acids (important for life!) in the presence of methane, and really hard to make them without, so the composition of the atmosphere is crucial.
A lot of the exciting chemistry happening in the interstellar medium that has important implications (much later on) for planetary habitability takes place on icy dust grains. Dust grains are important because they provide a surface onto which atoms and molecules can stick, move around, and react to form other compounds. The timescales are a lot longer than in the gas phase, where you basically get a split-second chance at a reaction during a collision. Dust grain-surface chemistry creates a lot of molecules we care about like oxygen, carbon dioxide, and methanol — we likely have dust to thank for life on Earth!
Dr. Tielens closed his talk with an encouragement for laboratory astrophysicists everywhere: everything you can study in the lab will be relevant somewhere in the universe — it’s your job to find out where!
Press Conference: Spiral Galaxies Near and Far (by Susanna Kohler)
This morning’s press conference threatened to make us all dizzy with four talks about spiral galaxies. We started with the nearest spiral galaxy — our own Milky Way — and then moved on to talk about these twisted galaxies more broadly.What’s going on at the very heart of our galaxy, in the central ~15 light-years? This region contains a tilted disk of gas and dust rotating counterclockwise around our galaxy’s supermassive black hole, Sgr A*. This ring is best observed at ~50 µm, a wavelength that can only be accessed from high altitudes; luckily, we’ve got SOFIA, our favorite flying observatory, on the job.
SOFIA’s HAWC+ instrument obtained both images and polarimetry — measurements of magnetic fields — of the central ~15 light-years of the galaxy. C. Darren Dowell (Jet Propulsion Laboratory) showed us the results: a spectacular map of the streamlines tracing the magnetic field within the heart of our galaxy (see the cover image at the top of the page). HAWC+’s measurements reveal a beautiful spiral structure to the dust that indicates the magnetic field is strong enough to channel material into orbit around the black hole. This subtle redirection may be enough to explain why our black hole is unusually quiet: magnetic fields might be preventing Sgr A* from getting a proper meal! Press release
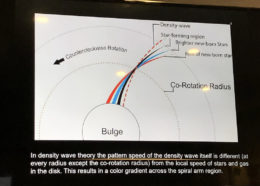
Spiral arms will have different apparent angles at different wavelengths as stars propagate away from spiral density waves.
The next two speakers both described tests of the dominant theory for what causes spiral structure in galaxies: density wave theory. According to this model, density waves propagate through the disk of a galaxy. Gas and stars don’t remain stationary relative to these spiral waves, though; instead, their rotation speed is set by their distance from the center of the galaxy, and this speed matches the pattern speed of the density wave only at one location, known as the co-rotation radius. Inside this radius, newly formed stars caused by compression of the gas will disperse ahead of the density wave; outside of the co-rotation radius, they trail behind the wave. According to the density wave theory, the shape of a spiral arm will therefore look different at different wavelengths: bluer wavelengths will trace the younger stars distributed close to the density wave, appearing as a more radial arm, whereas redder wavelengths will trace the older stars that have trailed further ahead of and behind the density wave, appearing as a more angled arm.
To test the picture of density wave theory, a team of scientists looked for these differences in arm shape across different wavelengths for ~30 galaxies. Daniel Kennefick (University of Arkansas) presented the results: the arms were indeed angled differently at different wavelengths, exactly as predicted by density wave theory. Kennefick remains cautious, however: this seems like a triumph for the density wave model, but other research groups have found opposing results. As always, more work remains to be done!
Shameer Abdeen (University of Arkansas) and collaborators took a different approach to test density wave theory: they attempted to measure the co-rotation radius for a sample of ~20 spiral galaxies. They did this by tracing out the galaxy’s spiral arms at different wavelengths, and then identifying the location where these arms crossed over one another. The good agreement of their results with other, independent measures of the co-rotation radius provides additional confirmation that density wave theory is a solid model.
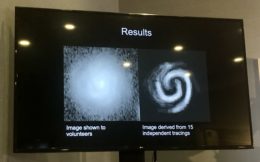
In the Spiral Graph citizen science project, volunteers trace out the spiral arms of galaxies. These tracings are stacked to produce a final map of a galaxy’s arms, which can then be used in the hunt for intermediate-mass black holes.
Abdeen and collaborators were able to trace out the spiral arms of their sample by hand, but what do you do if you have a sample of galaxies larger than ~20? Patrick Treuthardt (North Carolina Museum of Natural Sciences) has the answer: enlist the help of citizen scientists! Since computers have a hard time picking out spiral arms in low-contrast images, Treuthardt and collaborators looked to humans, who are inherently quite good at identifying patterns — even in faint images. To enlist enough humans to trace the spiral arms of a sample of thousands of spiral galaxies, the group set up a citizen science project based on the Zooniverse platform: Spiral Graph. In this project, anyone can go look at images of galaxies and mark where you think their spiral arms lie. Tracings of multiple citizen scientists are then averaged to produce a map of each galaxy’s arms.
Beyond being a fun way to kill time, this exercise has a purpose! Once we’ve mapped the arms of these galaxies, we can easily estimate their pitch angles. Intriguingly, how tightly these arms are wound correlates with the mass of the black hole hosted at the center of the galaxy. By selecting galaxies with very loose spirals, the team hopes to identify those galaxies most likely to host elusive intermediate-mass black holes (IMBHs). Astronomers only know of a dozen or so confirmed IMBH candidates thus far — so go do some spiral tracing and contribute to the discovery of more!
Plenary Lecture: The Tools of Precision Measurements in Exoplanet Discovery and Characterization: Peeking under the Hood of the Instruments (by Kerry Hensley)
Suvrath Mahadevan (Penn State University) gave the audience a peek “under the hood” of the highly precise instruments bringing us exoplanet detections and atmospheric characterizations. Thanks to missions like Kepler and the Transiting Exoplanet Survey Satellite (TESS), we’ve discovered thousands of exoplanets, with many more on the way. As a result, some of our focus has shifted from detection to characterization — we want to know what’s in the atmospheres of these planets and whether they might be habitable or not.
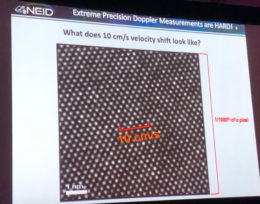
A depiction of what a shift corresponding to a velocity of 10 cm/s looks like on a detector. The silvery dots are individual silicon atoms.
What will it take to detect small, rocky planets and learn about their atmospheres? Really, really, really precise instruments. Currently, we can measure radial velocities of about 1 m/s. This is pretty good, but we’d like to get down to the tens-of-centimeters-per-second or centimeters-per-second level to detect lots of Earth-mass planets around faraway stars. That’s easier said than done, though! A velocity of 10 cm/s corresponds to a shift of just a few lattice-spacings on a detector — that’s 1/6000 of a 10-micron pixel!
What do we need in order to get down to that level of precision? A lot of things need to come together to make it happen: a really good spectrograph, a high-performance detector, a way of calibrating the instrument (e.g. laser combs), precise temperature and/or vacuum control of the environment that the instrument and detector are housed in, and an excellent data reduction pipeline, to name just a few.
Mahadevan highlighted a couple of current instruments: the Habitable Zone Planet Finder (HPF) on the Hobby-Eberly Telescope, and NEID, which will be installed on the WIYN telescope at Kitt Peak. HPF has given us the highest-precision near-infrared radial velocities ever reported. (As an aside, things get really interesting in the infrared: the act of reading out the detector warms it enough that the detector itself becomes a source of noise. There are also crystalline lattice defects that cause shifts in the quantum efficiency of the detector at sub-pixel scales, which can’t be removed through flat-fielding.)
Let’s say we can overcome these issues and build spectrographs and detectors capable of detecting the subtle shift in spectral lines corresponding to velocities of ~10 cm/s. We still have a problem: getting our instruments down to 10 cm/s won’t help us if we can’t disentangle the competing effects of transiting planets and solar activity. So, there’s still plenty of work to be done on the science side as well as the instrumentation side, both of which will help us in our quest to track down Earth-like planets.
Press Conference: More Sun & More Milky Way (by Susanna Kohler)
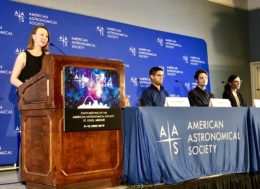
The afternoon press conference presenters are ready to go under the direction of AAS Media Fellow Kerry Hensley!
This afternoon’s press conference was hosted by AAS Media Fellow and astrobiter Kerry Hensley, and it promised more exciting results from our nearest star and galaxy.
First up, Kevin Reardon (National Solar Observatory) presented observations of the Sun from the millimeter/submillimeter powerhouse ALMA. The wavelengths probed by ALMA’s 64 antennae provide views of different layers of the Sun’s chromosphere, the atmospheric layer sandwiched between its cooler surface (the photosphere) and its hotter outer atmosphere (the corona). As “the gateway to the corona”, the chromosphere may eventually provide us with information about how heat is transferred from deep in the Sun’s interior to its outermost layers — if we can understand observations of it! Reardon and collaborators showed that ALMA’s 3.1-mm images of the Sun showed striking similarities to the more easily obtained optical images made using the Hα emission line (656.3 nm). This discovery will help us to better interpret Hα images in the future and further explore the layers of our Sun.
Next, Loren Matilsky (University of Colorado) presented the results of some heavy computer lifting. Since we can’t dive into the Sun and measure its magnetic fields, he reasonably points out, we have to make do with the next best thing: detailed computational models. Matilsky and his advisor Juri Toomre ran a massively parallel, fully 3D computer simulation of the Sun’s magnetic field evolution to understand what’s going on beneath the Sun’s surface and how that might drive the observed 11-year solar cycle. They found that a magnetic dynamo is generated to the north and south of the equator, migrates toward the equator, and is then reset — following the same pattern that we observe in sunspots. But in addition to this expected churning, Matilsky and Toomre found odder behavior: a wreath of magnetic field wandered back and forth between hemispheres, occasionally reversing polarity on a much longer cycle than the normal 11-year solar cycle. Is this a fluke of the simulation, or could this actually be representative of the behavior of magnetic fields beneath the Sun’s surface? Matilsky and Toomre aren’t sure yet, but they do note that the timing and behavior of this wandering dynamo is consistent with past observations that sometimes show heavier clustering of sunspots in one hemisphere for a while, and then in the other. Press release
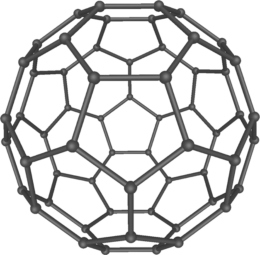
Model of the structure of a buckyball. [Mstroek]
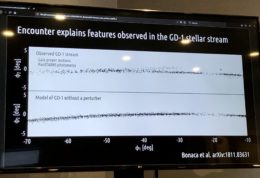
Can you spot the differences between the observations of the stellar stream GD-1 (top) and an unperturbed model (bottom)? Something massive appears to have passed through this stream, generating the gap and the small spur of stars.
The last speaker of the conference took us even further away, to the outskirts of the galaxy. There, stellar streams orbit within the Milky Way’s halo — and Ana Bonaca (Center for Astrophysics) and collaborators think these streams might reveal clues about the nature of dark matter. Stellar streams are thought to be created when stellar clusters in our halo are disrupted, drawing out long tails of stars that then orbit the Milky Way. The longer the tail, the longer the stream has likely been in existence. Bonaca reported on a study of one particular long stream of stars, GD-1, which she and collaborators analyzed in detail using data from PanSTARRS and Gaia. The team found that, upon closer inspection, the stream isn’t the smooth, continuous line of stars it appears to be. Instead, it has been perturbed: there are gaps and a small spur of stars mid-stream. Based on the team’s models, the most likely perturber was a compact object of around 5 million solar masses — yet such a perturber is nowhere to be seen. Could this perturber be a contributor to dark matter? Bonaca and collaborators plan to examine more streams to see if GD-1 is a fluke or a sign of more to come. If other streams show similar perturbations, we may be able to use these tracers to home in on dark matter models and explain where the invisible 90% of our galaxy is hiding.
Plenary Talk: Sexual Harassment – Changing the System (by Susanna Kohler)
In a replacement talk for the planned plenary — which Betsy Mills was unfortunately unable to give today — AAS Vice President Joan Schmelz (Universities Space Research Association) provided insightful and much-needed insights into how we can help change the system that allows sexual harassment to occur in astronomy.
In the wake of three very public sexual harassment cases in the field, Schmelz wondered what could be done to shift the burden from the vulnerable to the powerful members of the astronomy community. Since then, she has worked in her role as the former chair of the Committee for the Status of Women in Astronomy to identify ways we can shift the culture of the field.
Schmelz has since delivered talks at numerous institutions discussing ways that bystanders can move toward becoming allies and advocates. Her first recommendation is simple: each of us can start by moving one step up in the advocacy axis (see her slide at right). That means that if we’ve been the person telling sexist jokes, we should stop doing that. If we’ve been the person laughing at the jokes, we should stop laughing. If we’ve been a silent bystander before, next time we should speak up. Simple words such as “I disagree,” can shut down offensive conversation effectively. And, as with anything, practice makes perfect! Speaking up may not be easy at first, but it becomes easier with practice.
Schmelz addressed actions that can be taken at increasingly higher levels of leadership within an institution to change the culture around sexual harassment in the field. One interesting point she brought up is that faculty at many universities are mandatory reporters: they are legally required to (non-anonymously!) report cases of sexual harassment to the Title IX office or another campus authority. The Title IX office isn’t there to protect you, it’s there to protect the university, Schmelz points out. She argues that departments need to clearly present information like who is a mandatory reporter, and where targets of harassment can go on campus to receive guidance while keeping their identity anonymous.
The final topic Schmelz addressed is that of serial harassers. Targets of harassment are often — understandably — unwilling to report, fearing a he-said, she-said scenario. But what if it’s he-said, she-said, she-said, she-said? In the fluid environment of academia where people frequently move between institutions, the target of a serial harasser may have no idea that their experience wasn’t unique. An information escrow addresses this problem.
In an information escrow, an individual can file a report that will remain confidential unless and until a second report is filed against the same harasser. At that time, both filers are notified and can make the decision of how to proceed — whether they wish to have contact with one another, file a Title IX complaint, wait for a third case, or pursue another course of action. Schmelz pointed out that such an information escrow would be most effective if managed by the astronomy community as a whole, so that records remain intact as individuals move between institutions. She welcomed the community to take collective action to make such an escrow happen.
It’s clear that the astronomy community as a whole benefits when everyone is able to work to the best of their potential in a positive department climate. So why wouldn’t we all come together to change the system? It’s time that we each do our part.
Town Hall: The National Academies of Sciences: The Astronomy and Astrophysics Decadal Survey: 2020 (by Susanna Kohler)
They promised that we’d have town halls as part of Astro 2020, and here we are! The Astro 2020 Decadal Survey is a process during which the astronomy community assesses the current state of the profession and makes recommendations for what missions and projects should be prioritized over the coming decade. This evening Robert Kennicutt, co-chair of the Astro 2020 survey committee, presented an update on the current state of the survey.
In a quick overview, Kennicutt reminded us of what the committee has been tasked to do. Notably, in addition to apprising stakeholders of the state of the field and making recommendations, the survey committee must also temper their recommendations with what Kennicutt called “what-if” scenarios — i.e., they should develop contingency recommendations for cases where a proposed mission runs over budget or behind the proposed timeline.Where are we currently in the process? White papers have been solicited from the community, the survey committee has been selected, and the committee is currently building topical panels to review white papers and advise the survey committee. Panel deliberations will be conducted in late 2019, and survey-committee deliberations and report-writing will occur in spring and summer 2020.
We’re early in the game yet, but so far it looks like community involvement is high! As an example, the number of received science white papers is up by ~75% compared to the previous decadal survey, reports Kennicutt. The call for Activities, Projects, or State of the Profession Consideration white papers will be open for another month, and after that time the community can look for a few more town halls — including one at the winter AAS meeting in Honolulu, HI — as a means of keeping up to date with Astro 2020.
1 Comment
Pingback: Daily Study Log (2019-06-13) - Study Astrophysics