Editor’s note: Astrobites is a graduate-student-run organization that digests astrophysical literature for undergraduate students. As part of the partnership between the AAS and astrobites, we occasionally repost astrobites content here at AAS Nova. We hope you enjoy this post from astrobites; the original can be viewed at astrobites.org.
Title: First measurement of the Hubble constant from a dark standard siren using the Dark Energy Survey galaxies and the LIGO/Virgo binary–black–hole merger GW170814
Author: Marcelle Soares-Santos, Antonella Palmese, et al. (DES, LIGO, and Virgo collaborations)
First Author’s Institution: Brandeis University (M. S.-S.), Fermi National Accelerator Laboratory (A. P.)
Status: Published in ApJL
Disclaimer: The author of this Astrobites post is a member of the Dark Energy Survey but researches a different topic and did not take part in this analysis.
Nearly all of the galaxies we observe in the night sky are rushing away from us. Only the Andromeda galaxy is moving toward us — we are trapped in a gravitational dance that will end in a major collision about 4.6 billion years from now. The remainder of galaxies are receding due to the expansion of the universe. But how fast are the rest of the galaxies flying away from us? This is actually a difficult question to answer, partly because it is difficult to accurately measure distances across the universe. Today’s paper details a new method to measure how quickly the universe is expanding using the gravitational-wave (GW) signals from binary black hole collisions.
The Sounds of the Universe
Gravitational waves are ripples of spacetime itself, analogous to sound waves traveling through the air. They are generated in violent collisions between compact objects like neutron stars and black holes. The LIGO and Virgo collaborations have detected 11 such collisions, ten of which have been the collisions of two black holes (see Figure 1). The frequency and amplitude of the GWs, or the pitch and volume of the “sound,” encode information about the mass of the merging system and how far away it is. Exactly how the signal evolves tells us everything we need to know about the gravitational “brightness,” or luminosity, of the event. By comparing the measured amplitude to the calculated amplitude, we get a precise distance to the source. The ability to do this with GW signals has earned their sources the name “standard sirens.”
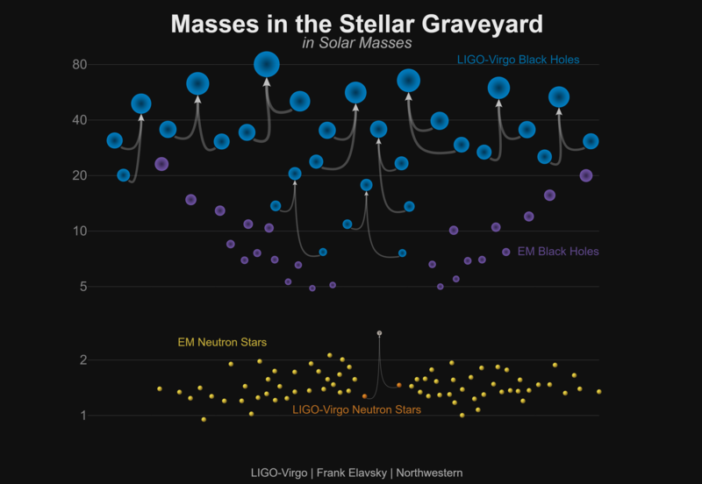
Figure 1. Our current knowledge of the end states of massive stars, namely black holes and neutron stars. Because the GW signals are so precisely tied to the properties of the system, we can determine the masses of the initial objects before merger in addition to the mass of the final object after merger. [LIGO-Virgo/Frank Elavsky/Northwestern U.]
On the other hand, standard sirens with electromagnetic counterparts don’t rely on the cosmic distance ladder and so offer an independent way to measure H0. In this case, the electromagnetic signal pins down the host galaxy’s location, which identifies the redshift of the signal and thus its velocity. At the same time, the GW signal gives the precise distance to the source. In fact, this has already been done using the binary neutron star merger GW170817. But we need many more than one binary neutron star event to truly pin down H0 (Figure 3), and ten of the 11 LIGO/Virgo events have not had accompanying EM signals. Today’s paper shows that there is still a way to calculate H0 from these events!
Listening in the Dark
The authors of today’s paper report the first measurement of H0 using the “dark” siren GW170814, a GW signal from two colliding black holes with no accompanying electromagnetic radiation. Recall from Hubble’s Law that we need a distance and a redshift to calculate H0 — but to determine a redshift, we need to know what galaxy the source was located in. That’s a hard thing to determine with no electromagnetic counterpart signal. The probability maps produced by LIGO/Virgo for the on-sky location of the GW signal can encompass a large area containing tens of thousands of galaxies at as many different redshifts!
GW170814 happened to fall smack in the middle of the Dark Energy Survey (DES, see Figure 2). DES has produced exquisite galaxy maps of a quarter of the Southern Hemisphere sky, complete with estimated redshifts calculated from the coarse “spectrum” of DES’s five wavelength filters. Soares-Santos, Palmese, et al. devised a statistical analysis that selected potential host galaxies from DES’s galaxy maps using the LIGO/Virgo maps and calculated what H0 would be for each in turn.

Figure 2. The LIGO/Virgo highest probability region for where GW170814 originated from, overlaid on the DES survey area. [Dark Energy Survey Collaboration]

Figure 3. Comparison of values of H0 calculated from the CMB (Planck, dark blue), supernovae (ShoES, light blue), the binary neutron star event (GW170817, grey), and the dark siren (DES GW170814, red). With ~100 GW events, we will approach the sensitivity of the traditional electromagnetic measurements, giving an independent measurement of H0. [Soares-Santos et al. 2019]
About the author, Stephanie Hamilton:
Stephanie is a physics graduate student and NSF graduate fellow at the University of Michigan. For her research, she studies the orbits of the small bodies beyond Neptune in order learn more about the Solar System’s formation and evolution. As an additional perk, she gets to discover many more of these small bodies using a fancy new camera developed by the Dark Energy Survey Collaboration. When she gets a spare minute in the midst of hectic grad school life, she likes to read sci-fi books, binge TV shows, write about her travels or new science results, or force her cat to cuddle with her.
1 Comment
Pingback: AAS Nova – New