Astronomers still don’t fully understand how planets form, especially ultra-dense, iron-rich planets like Mercury. How do trillions of tiny dust grains clump together to make pebbles, planetesimals, and eventually the cores of rocky planets?
Arrested (Dust) Development
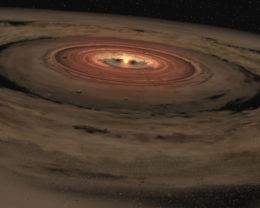
Protoplanetary disks are composed of gas and dust, as this artist’s impression shows. How the tenuous gas–dust blend forms planets is still an open question. [NASA/JPL-Caltech]
If the bouncing barrier stops dust grains from getting progressively larger, how do planets ever manage to form? One possibility is that the presence of an external magnetic field enhances the intrinsic magnetization of the iron in dust grains, which increases the attractive forces between them, making them “stickier.” If magnetic fields can help dust aggregates grow to centimeter size, other growth mechanisms like the streaming instability — a clumping mechanism caused by gas drag in a disk — can take over from there.
From simulations and observations, we expect the inner regions of protoplanetary disks to have strong magnetic fields — somewhere between 1 and 100 mT. How strongly do magnetic fields of this scale affect the growth of iron-rich dust aggregates?
Levitation and Magnetization
Maximilian Kruss and Gerhard Wurm (University of Duisburg-Essen, Germany) used laboratory experiments to explore the effects of magnetic fields on the bouncing barrier. Kruss and Wurm used a heat lamp to levitate micron-scale iron–silicate dust grains above a glass lens, which allows them to move and collide freely.
With no external magnetic field, the bouncing barrier stops the grains from growing larger than 2 mm. The authors find that for dust composed of equal amounts of iron and silicate, the bouncing barrier begins to shift when the external magnetic field strength reaches 2.2 mT. The size of the aggregates increases with increasing magnetic field strength, reaching 6 mm in length when the maximum field strength for the experimental setup (7 mT) is applied.
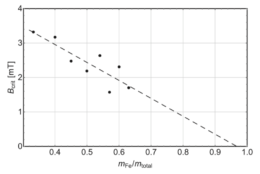
The minimum magnetic field necessary to shift the bouncing barrier as a function of iron mass fraction. Grains with an iron mass fraction below 0.33 didn’t form grains, while those with an iron mass fraction above 0.63 were too dense to be levitated. [Kruss & Wurm 2018]
The Missing Link?
The magnetic field strength necessary to shift the bouncing barrier could be even lower; if dust grains in protoplanetary disks are more iron-rich than those used in this experiment, the required field strength could be below 1 mT — well within the expected range for protoplanetary disks.
It isn’t yet known what proportion of dust grains can be expected to be enriched in iron, but this experiment clearly shows that the presence of an external magnetic field encourages iron-rich dust grains to grow larger.
If magnetic fields can suppress the bouncing barrier so that the grains grow large enough for the streaming instability to take over, we may be able to explain how planet formation gets going on the very smallest scales, especially for iron-rich planets like Mercury.
Citation
“Seeding the Formation of Mercurys: An Iron-sensitive Bouncing Barrier in Disk Magnetic Fields,” Maximilian Kruss and Gerhard Wurm 2018 ApJ 869 45. doi:10.3847/1538-4357/aaec78
2 Comments
Pingback: Can Magnetic Fields Help Planetesimals Form? – New
Pingback: Daily Study Log (2019-02-17) | Study Astrophysics