Editor’s note: Astrobites is a graduate-student-run organization that digests astrophysical literature for undergraduate students. As part of the new partnership between the AAS and astrobites, we will be reposting astrobites content here at AAS Nova once a week. We hope you enjoy this post from astrobites; the original can be viewed at astrobites.org!
Title: The Asteroid Belt as a Relic From a Chaotic Early Solar System
Authors: Andre Izidoro, Sean N. Raymond, Arnaud Pierens, Alessandro Morbidelli, Othon C. Winter, David Nesvorny
First Author’s Institution: Laboratoire d’astrophysique de Bordeaux, Pessac, France
Status: Accepted to ApJ
The puzzling architecture of the Solar system has long been a headache for planetary dynamicists. We can sort of divide its structure (cover image, not to scale) into several zones. First, the terrestrial planets Mercury, Venus, Earth and Mars, which are divided from the gas and ice giant planets by the asteroid belt. Beyond the ice giants there is the Kuiper belt, which spans out to very large distances from the Sun. One longstanding conundrum in this ordering is the relatively small mass of Mars and existence of the asteroid belt in between Mars and Jupiter. It shouldn’t be there, and Mars should be way bigger. In fact, Mars is only 10% of the mass of Earth and therefore seems to have never accreted enough material to become a fully fledged planet. In planet formation, Mars-sized objects are usually termed “planetary embryos”, as we think this is the intermediate stage of a planet’s growth.
Sailing adolescent Jupiter to blame?
To explain the apparent dip in the mass-distance relation of our solar system (increasing mass with planetary distance from the Sun until Jupiter, and then decreasing again), planetary scientists developed a model deeply rooted in dynamical principles: the “Grand Tack”. The idea of the model is that Jupiter, directly after its formation out of the early Solar nebula at ~3.5 AU (AU = distance Sun–Earth), migrated toward the Sun, became “tacked” on at ~1.5 AU and then migrated outward again to its current positon at 5.2 AU. By doing so it depleted the mass concentrated at the locations of nowadays Mars and the asteroid belt, and thus Mars was never able to grow bigger. It also successfully explains some other issues, such as the inclinations and excitations of asteroids and the transition from water-poor to water-rich asteroids in the middle of the belt.
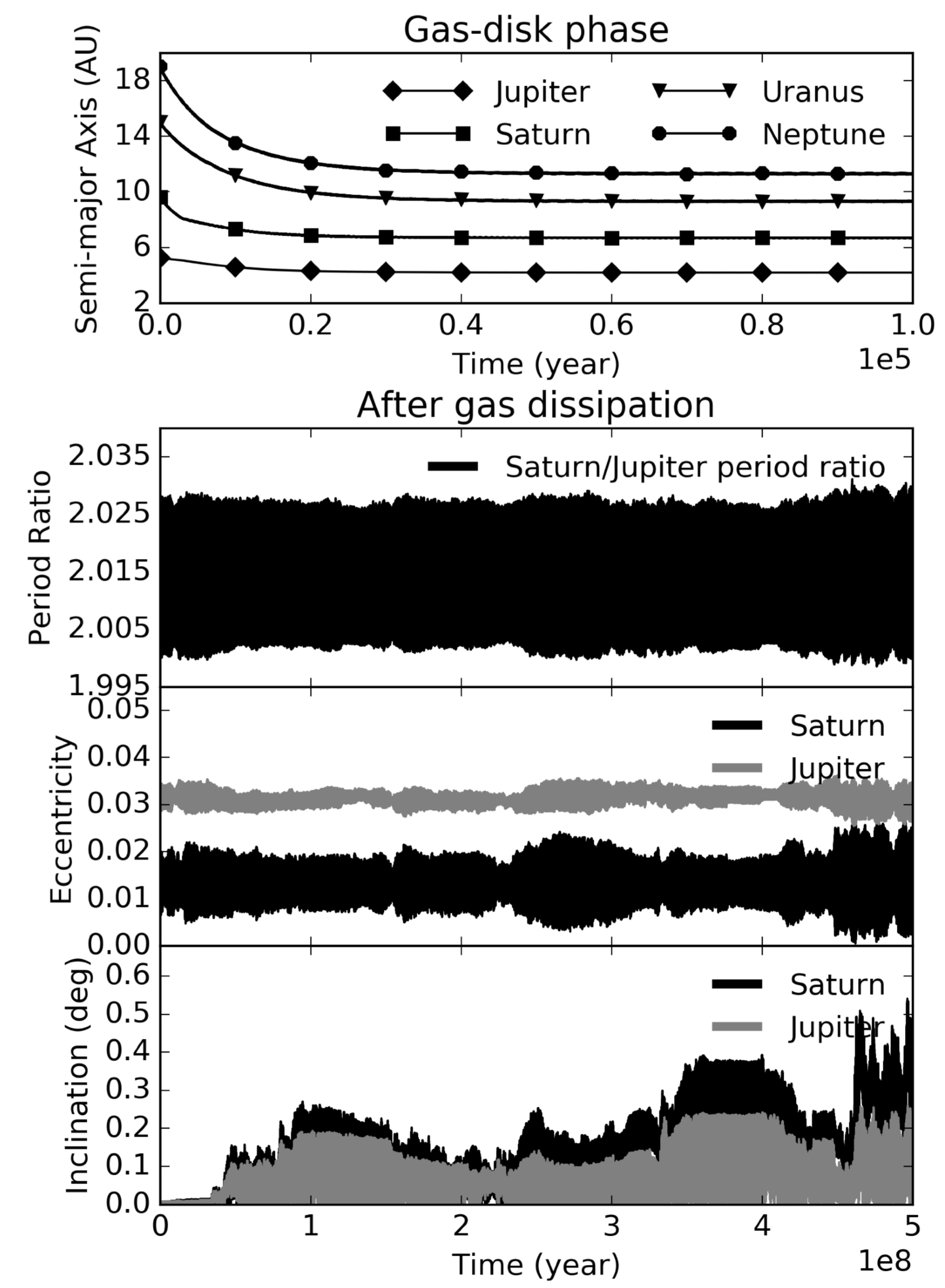
Chaos in the giant planets’ orbits and their long-term stability. During the gas-disk phase the giant planets migrated inward toward the Sun and are eventually locked in so-called mean-motion resonances. After the gas dissipation, Saturn and Jupiter orbit in chaotic but stable orbits. [Izidoro et al. 2016]
A more “primordial” origin
Alternatively, the mass-depletion at Mars’ location could have very early roots, even before (or during) the formation of Jupiter, caused by microphysics in the disk, as was explained in this Astrobite about the pile-up and evolution of dust. Here, the initial small dust grains in the disks did not accumulate everywhere in the disk and start to form planets. Instead, they end up only in specific regions and thus planet formation is concentrated in some narrow zones. In our case, this could be in two locations: one in the inner region, where the terrestrial planets reside nowadays, and one in the outer region, possibly around the region of Jupiter (more details given in this recent review paper). Such a scenario however poses another problem. The Grand Tack scenario from above easily explains the current relatively high inclinations and excitations of the asteroids in the belt: Jupiter drops by and gives everyone a huge gravitational swing due to its enormous mass. In the other scenario this isn’t the case — so we would nominally expect the asteroids to remain in relatively calm and low-excitation orbits.
But Andé Izidoro and collaborators think differently. They propose that instead of migrating (like in the Grand Tack model), Jupiter and Saturn were on chaotic but stable orbits. This scenario is illustrated in the figure above, which shows how the gas and ice giants evolved over time in such a configuration. Essentially what happens is that all the planets roughly formed at approximately where they are today. However, as our early solar system was subject to dynamic evolution (we possibly lost some planetary embryos early on), the orbits of Jupiter and Saturn moved continously and chaotically through the disk (though not nearly as dramatically as in the Grand Tack scenario). Thus, Jupiter came close to the asteroid belt again and its gravity excited the asteroids. You’ll probably ask yourself now where the great difference lies. This is highlighted in the figure below, where the deviations between the Grand Tack and the “chaotic motion” pictures become readily apparent.
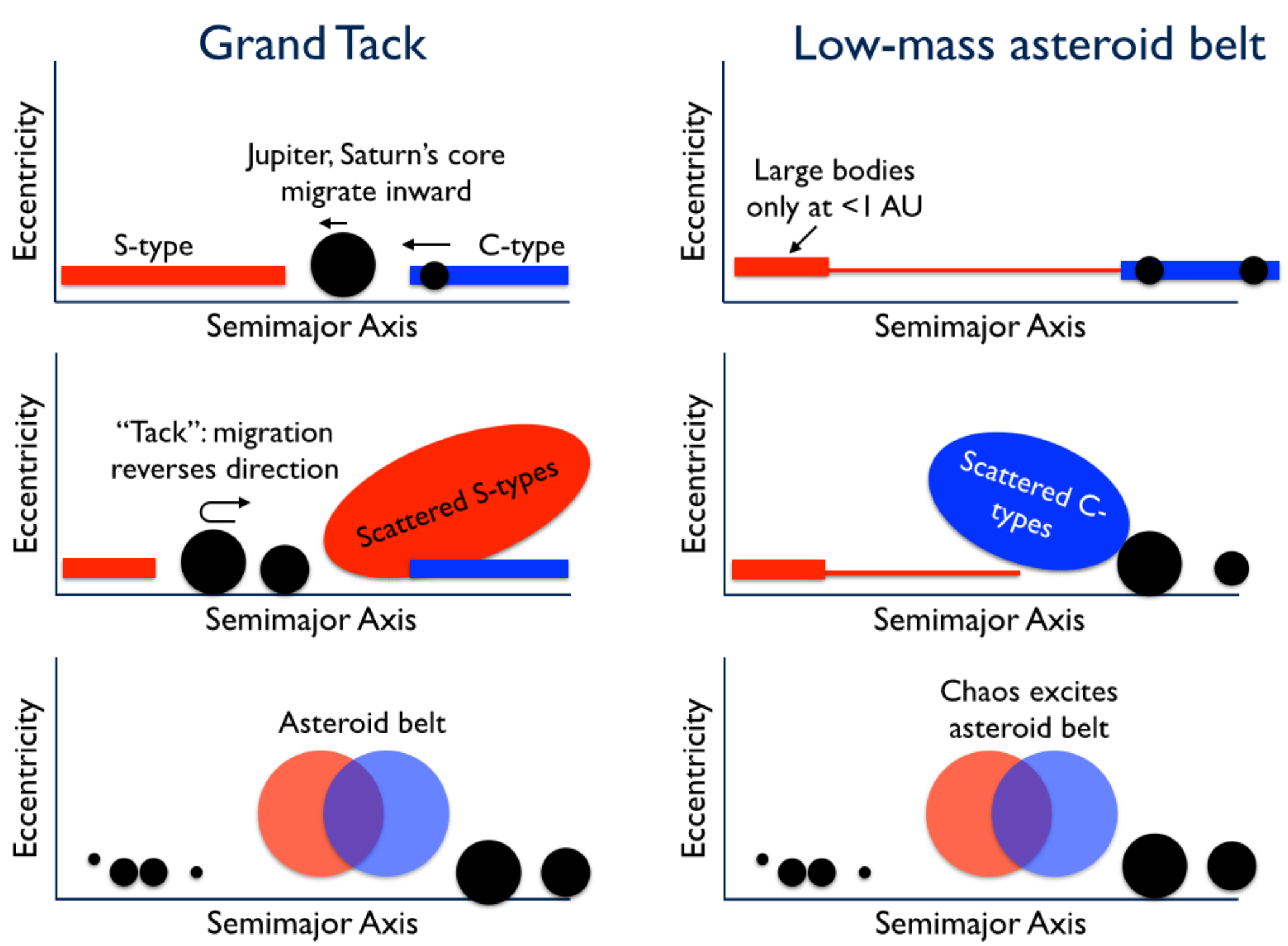
In the Grand Tack model, Jupiter is formed within a sea of primordial planetesimals/asteroids (which are divided into S- and C-types: water-poor and water-rich). It migrates inwards and then outwards, and scatters some of the outer asteroids to their current locations. In the “low-mass asteroid belt”/”chaotic motion” model the S- and C-type deviation and the mass-depletion at around Mars’ current location is primordial. Jupiter and Saturn form later and evolve in chaotic but stable orbits, which scatters some of the outer asteroids inside. [Morbidelli & Raymond, 2016, arXiv:1610.07202]
What now?
Instead of one we have now two models, both of which can give us an answer to why our solar system’s architecture looks like it does today. Fortunately, the two models make different predictions about how the dynamics evolved in the disks. In the coming years we may thus be able to distinguish between them by testing their differences, and we may be able to get deeper insights into how our home in the universe evolved into how it looks today.
About the author, Tim Lichtenberg:
I am a graduate student at ETH Zurich in planetary astrophysics. By combining astro- and geophysical numerical approaches I try to understand the influence of star-forming environments on the formation of terrestrial planets. Occasionally, when I am not at the bus swinging back and forth between the institutes, I enjoy doing sports or become involved in science outreach projects.
5 Comments
Pingback: Jangan Lupa Kita Ada Di Mana | Renungan Renungan
Pingback: Skala Tata Surya | Renungan Renungan
Pingback: This Website Calculates Your Age And Birthday On Different Planets - Viral Bake
Pingback: Osončje – H5p
Pingback: Our Solar System, Amid A Whole Universe - MozartCultures