Editor’s note: Astrobites is a graduate-student-run organization that digests astrophysical literature for undergraduate students. As part of the partnership between the AAS and astrobites, we occasionally repost astrobites content here at AAS Nova. We hope you enjoy this post from astrobites; the original can be viewed at astrobites.org.
Title: A Triple AGN in a Mid-Infrared Selected Late Stage Galaxy Merger
Authors: Ryan W. Pfeifle, Shobita Satyapal, Christina Manzano-King, Jenna Cann, et al.
First Author’s Institution: George Mason University
Status: Published in ApJ
What Are AGN and Why Do I Care?
An AGN, or active galactic nucleus, is the epitome of a fascinating and truly awesome astrophysical object. An AGN consists of a black hole that happens to be surrounded by gas and dust that is close enough to form an accretion disk and fall into the black hole. As a result, the black hole (now AGN) forms these enormous, extremely bright, and hottt jets. AGNs are quite useful for those interested in learning more about black holes (who isn’t?), but black holes on their own don’t emit any sort of radiation. It’s only when they come in contact with or are in front of other, directly detectable things that we can then indirectly learn about these incredible astrophysical phenomena.
Astronomers are particularly interested in supermassive black holes, which seem to be in the center of the vast majority of galaxies. Supermassive black holes also seem to co-evolve with their host galaxy, at least according to the most popular theory of cosmology: ΛCDM. It’s quite interesting to think that a single supermassive black hole in the center of the galaxy, while very massive for a single object, could also potentially govern the evolution of its much larger and more massive entire host galaxy. For example, the supermassive black hole in the center of the Milky Way is about 3 million solar masses and just about a light-minute in diameter — but compare that to the Milky Way itself, which is 150 billion solar masses and over 50,000 light-years wide. How could that relatively small black hole have such an impact on an entire galaxy?Since AGNs are super bright, astronomers can detect them from very far away. This allows us to explore how supermassive black holes affect galaxy evolution across cosmic time. AGNs only exist where there is an excess amount of dust and gas in a galaxy. Nowadays, when we look at nearby galaxies, they are lacking in dust and gas compared to galaxies at a redshift of z = 2. Examining galaxies at a redshift of z = 2 mean that we are looking back to a time when the universe was only about a quarter of its current age. Back then, galaxies contained a TON of gas and dust that was used for star formation — and also as fuel for AGNs. This redshift is therefore a key time period that astronomers can probe to better understand how galaxies and their active supermassive black holes evolve to what they are today.
Where Does the Triple AGN Come In?
One of the main drivers of galaxy evolution is galaxy mergers. The math is simple: galaxy + galaxy = bigger galaxy. When two galaxies collide, the stars inside of them don’t run into each other, but the gas and dust in each galaxy do collide and interact, producing starburst events and, you guessed it, powering AGNs! So if we wanted to hunt down some AGNs, mergers are a fantastic place to start.
Cosmological simulations predict that ~16% of all mergers actually contain three galaxies — a triple merger. This offers up the possibility of a triple merging system where each of the galaxies involved in the collision has an active galactic nucleus. If we believe our modern theory of the universe (ΛCDM), triple AGN systems must exist, but since there is going to be a lot of dust in the system, they could be obscured behind thick sheets of dust. This possible obscuration — plus their relative rarity — makes these systems difficult to detect. Today’s paper offers up a proposed detection of such an object.The Detection of a Triple AGN
Detecting AGN is not always straightforward. The signals that AGNs produce are similar to other high-energy phenomena in the universe, so in order to be absolutely sure you have detected an AGN, you need to use the full electromagnetic spectrum. AGN flux peaks at X-ray wavelengths, so you definitely want to use the Chandra and NuStar observatories. AGNs also have a specific optical signature to look out for, so you can use a big ol’ optical telescope like the Large Binocular Telescope, and optical surveys like SDSS. And due to the dust surrounding the AGN, its original emission will be reddened, and even the dust itself can be visible in infrared wavelengths. Today’s paper utilized telescopes that operate in each of these wavelengths to confirm a triple AGN system: SDSS J0849+1114. (Radio is also useful, and the authors say that they are working on another paper that will include the radio data).
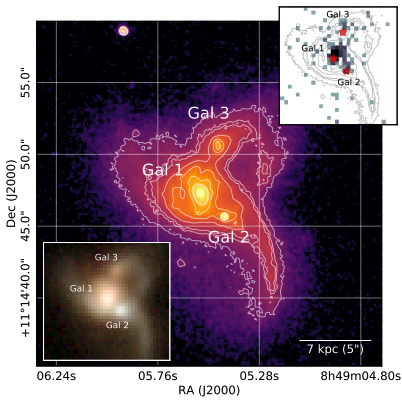
Figure 1: This figure shows the triple merger system: three galaxies, each of which host an AGN. The middle image is from the Hubble Space Telescope (infrared); the image in the upper right is from Chandra (X-ray); and the lower left is from SDSS (optical). [Pfeifle et al. 2019]
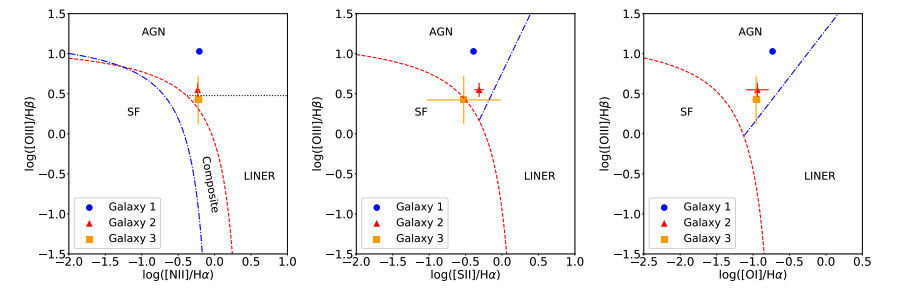
Figure 2: These plots show the line ratios of various elements. That basically means that we measure the emission we get at wavelengths that correspond to very specific atomic transitions. We can use that emission, and ratios of emission from different elements, to determine what kind of environment we are looking at. In these specific plots, the blue, red, and yellow shapes represent the centers of the three galaxies, and the markers can either lie in the AGN, SF (star formation), or LINER (low-ionisation nuclear emission-line region) category. We see here that the three line ratios that they explore suggest that each of the galaxies contain AGN. [Pfeifle et al. 2019]
About the author, Jenny Calahan:
Hi! I am a second year graduate student at the University of Michigan. I study protoplanetary disk environments and astrochemistry, which set the stage for planet formation. Outside of astronomy, I love to sing (I’m a soprano I), I enjoy crafting, and I love to travel and explore new places. Check out my website: https://sites.google.com/umich.edu/jcalahan
1 Comment
Pingback: Daily Log (2019-11-13) - Study Astrophysics