Being able to make precise measurements of distances and redshifts will help us understand how the universe is evolving. With the advent of gravitational wave observatories, we can make these measurements by using black holes in a very different way than before.
Standard Sirens
To measure how the universe is expanding, we need to simultaneously obtain the distances and redshifts to sources. When it comes to measuring large distances in space, astronomers have typically leaned on “standard candles” — objects whose intrinsic brightness is known. The dimmer a standard candle appears, the farther away it is.
Merging binary black holes (BBHs) can serve as standard candles, in a way. When compact objects like black holes merge, they produce gravitational waves, which can be picked up by observatories like the Laser Interferometer Gravitational-Wave Observatory (LIGO). The emitted gravitational waves have a characteristic energy, meaning that these mergers could be used to measure distances as “standard sirens”.
The trouble comes when trying to simultaneously measure the redshift of these sources. The gravitational wave detection on Earth gives us a mass measurement for the black holes that’s a combination of their redshift and their true masses in the source frame. If we know the true masses, we can disentangle these variables and determine the redshift. To achieve this, Will Farr (Stony Brook University and Flatiron Institute) and collaborators propose using a particular constraint on the masses of BBHs.
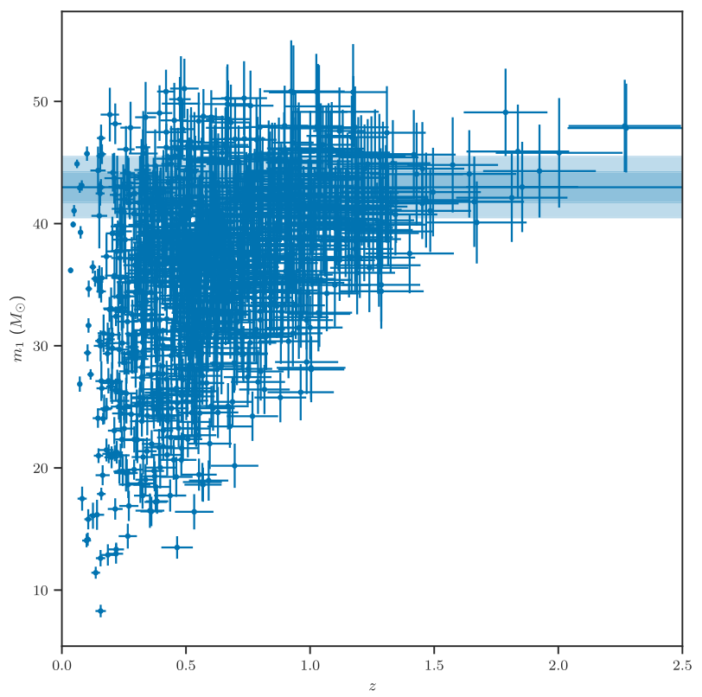
True black hole mass (not measured mass) versus redshift as obtained from one year of simulated BBH merger observations. The blue line indicates the maximum mass of black holes as set by PISNs, with the dark and light bars showing the confidence intervals. [Farr et al. 2019]
Capping Masses
When we model the population of merging black holes we’ve detected via gravitational wave observations, we see a drop-off in black hole mass above 45 solar masses. Farr and collaborators suggest this upper limit could be tied to one specific route of black hole creation: pair instability supernovae (PISNs).
Only massive stars can die as PISNs. In these events, the core of a star gets hot enough to allow electron–positron pairs to pop into existence, which lowers the star’s internal pressure enough for gravity to trigger the trademark explosion of a supernova. The remnants left behind by PISNs peak in mass around 45 solar masses.
By taking advantage of the mass scale imprinted on the population of BBH mergers by the PISN process, Farr and collaborators argue, we can extract redshifts from our detector measurements. Simulating 5 years of detections, the authors show that we could potentially constrain the Hubble parameter — our measurement of the expansion of the universe — at a specific redshift to within an impressive 2.9%.
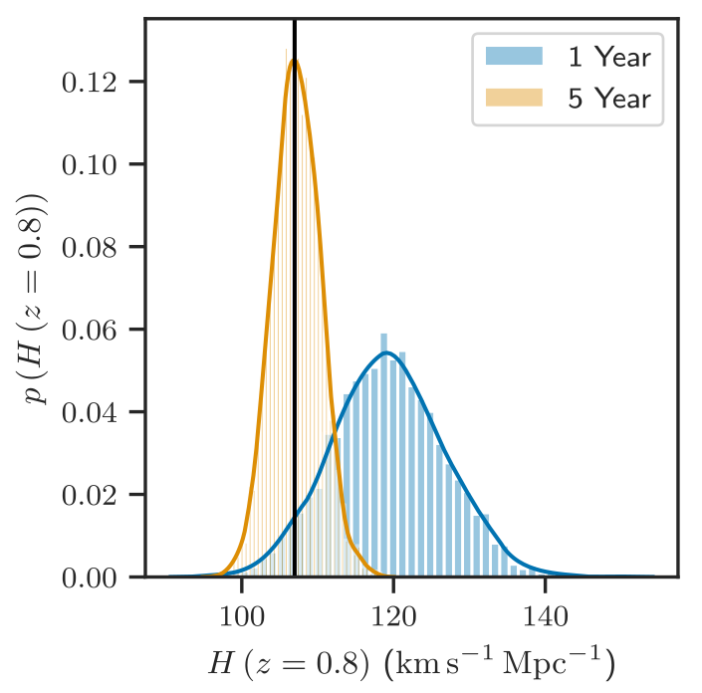
Distributions of the Hubble parameter at a redshift of 0.8 as estimated by one year of observations (blue) and five years of observations (orange). The true value of the Hubble parameter at that redshift is indicated by the black vertical line. [Adapted from Farr et al. 2019]
Paring Down Parameters
The authors find that BBHs are most useful for constraining the Hubble parameter at a redshift of z = 0.8 (redshifts that can be explored with the current capabilities of gravitational wave observatories are between z = 0 and 1.5). This is because at that redshift the models peg the uncertainty on the Hubble parameter at a minimum. Additionally, the uncertainty is halved when going from one year of observations to five years.
The authors note that a change of 1–2 solar masses in their maximum black hole mass does not change their results drastically. Their method would also work with a different maximum mass — so long as there is some mass scale, BBH mergers can be used to measure distances.
New gravitational-wave detectors will extend our sample of BBH mergers enormously. With a larger sample and a better understanding of the utility of black holes, we will be closer to pinning down the fate of the universe.
Citation
“A Future Percent-level Measurement of the Hubble Expansion at Redshift 0.8 with Advanced LIGO,” Will M. Farr et al 2019 ApJL 883 L42. https://doi.org/10.3847/2041-8213/ab4284
1 Comment
Pingback: Stellar Snacks or: How to Grow a Massive Black Hole through TDEs | astrobites