Editor’s Note: Astrobites is a graduate-student-run organization that digests astrophysical literature for undergraduate students. As part of the partnership between the AAS and astrobites, we occasionally repost astrobites content here at AAS Nova. We hope you enjoy this post from astrobites; the original can be viewed at astrobites.org.
Title: RUBIES: Evolved Stellar Populations with Extended Formation Histories at z ∼ 7 − 8 in Candidate Massive Galaxies Identified with JWST/NIRSpec
Authors: Bingjie Wang et al.
First Author’s Institution: Pennsylvania State University
Status: Published in ApJL
When JWST was launched, scientists were hopeful that the telescope would be able to answer hundreds of questions about the early universe. Luckily, it has been able to answer many of these questions. And even more luckily, observations of the early universe with JWST have been able to generate even more questions we don’t know the answers to!
One new conundrum scientists are running into is that it looks like some galaxies in the early universe have what we call evolved stellar populations. This means that even though these galaxies are only a couple hundred million years old (super young, right?), they already have ~100-million-year-old stars, meaning that these galaxies had to make a large amount of stars in only a short span of time. We’re not used to seeing this many stars form this fast — in fact, we expect that star formation should be slow this early in the universe. So how do we reconcile this?
The authors of today’s article look in more depth at the spectra of three such galaxies to figure out if these galaxies have really been forming stars for millions of years already. These three galaxies are located at redshifts of z = 6.7–8.4, which places them just 600 million years after the Big Bang.
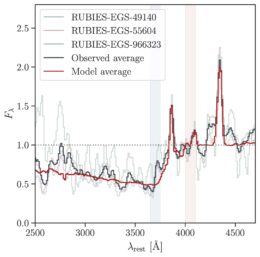
Figure 1: Balmer break at rest wavelength 3645 Angstroms (Å) observed in the galaxy spectra. The strength of the break (the relative flux difference before and after the break) is 1.71. [Wang et al. 2024]
Balmer Breaks Create Questions
First, the authors looked to confirm that evolved stellar populations do in fact exist in these galaxies. One foolproof way to do this is to look for a Balmer break in the galaxies’ spectra. The Balmer break comes from the atmospheres of older stars, indicating that the galaxy contains a population of older stars and has had little star formation for at least the past 100 million years. Excitingly, the authors confirm that there is in fact a Balmer break in the spectra of these three galaxies, as shown in Figure 1. Unfortunately, the Balmer break only tells you that there are older stars, not necessarily how old they are or how many of them there are.
Stellar Populations or Active Galactic Nuclei?
The authors then turn to understanding the finer details of the stellar population, specifically its formation history. The authors found evidence of broad lines in their emission-line spectra, signaling that there might be active galactic nucleus activity in at least two of these galaxies. Broad lines come from Doppler broadening, where photons emitted by an active galactic nucleus have a wide distribution of velocities, resulting in a broad emission line. However, the authors aren’t entirely ready to say conclusively that there are active galactic nuclei in the spectra.
In the local, low-redshift universe, we’re pretty good at understanding how these broad lines relate to active galactic nuclei because we have hundreds of spectra to test. But at high redshift, we don’t have a lot of data. We aren’t sure why supermassive black holes act the way they do early in the universe, and we don’t want to assume they act the same as the ones we observe in the local universe. So researchers have to rely on testing theoretical models against high-redshift galaxy observations.
To test how much of the light in these galaxies is coming from active galactic nuclei or evolved stellar populations, the authors test three different models: 1) The light is mostly from stars. 2) It’s from a mixture of stars and active galactic nuclei. 3) It’s mostly from active galactic nuclei. The authors create the model spectra using Prospector and compare the modeled spectra to the observed spectra to see how well they match.
Model Comparisons
The results of the modeling are shown in Figure 2. The most important thing the authors are testing here is what these different levels of contribution from the active galactic nucleus mean for the formation history of the stellar population. For instance, the model with the maximal stellar contribution means that the stellar population had to form earlier, because there need to be enough stars to create all the light seen. The minimal stellar model can form stars a bit later because the active galactic nucleus contributes more to the light seen, so you don’t need as many stars producing a lot of light. However, there is no way to verify which model is more accurate at this stage, so the authors turn to theorizing how each model could fit into the evolutionary stages of later galaxies.
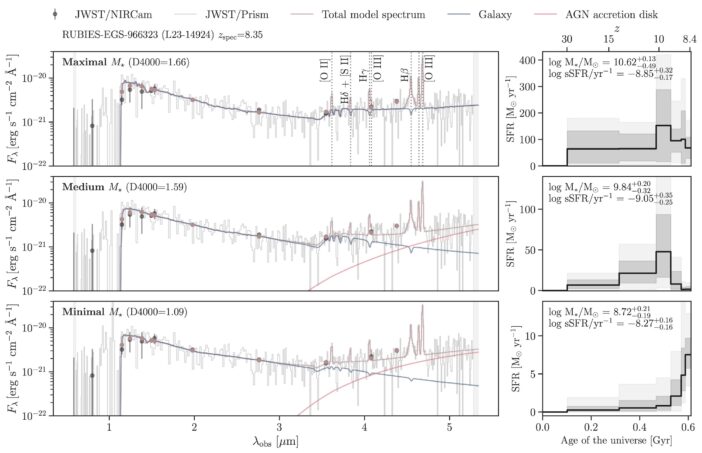
Figure 2: Left: Each model compared to the spectrum for one of the observed galaxies. Right: Predicted star formation rate as a function of time since Big Bang for each model. As seen, models with higher active galactic nucleus contribution predict later star formation. [Wang et al. 2024]
Progenitors of Massive Quiescent Galaxies?
To find out how their models fit into the evolution of galaxies, the authors then compare the different suggested star formation histories of the medium and maximal stellar contribution models with the projected histories of massive quiescent galaxies that exist at z ~ 3-5. These massive quiescent galaxies lack an explanation for how they got so massive yet have such slow star formation now. As can be seen in Figure 3, the star formation rate traced across the age of the universe for the massive galaxies (black, blue, green, and yellow distributions) matches much better in the maximal stellar contribution model. The authors posit that these galaxies could create a lot of stellar mass in a short time, then have their star formation slowed greatly to become these massive quiescent galaxies. With this explanation, the authors note that these galaxies would be a relatively small fraction of all the galaxies in the early universe.
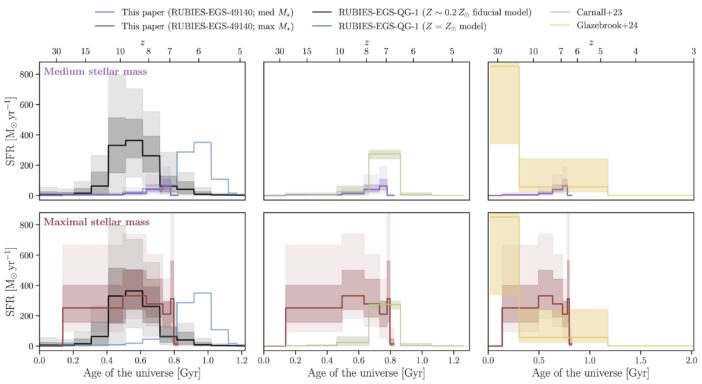
Figure 3: Comparison of medium (purple) and maximal (red) stellar models with the projected formation histories of a sample of massive quiescent galaxies at z ~ 3-5. [Wang et al. 2024]
Original astrobite edited by Amaya Sinha.
About the author, Caroline von Raesfeld:
I’m a second-year PhD student at Northwestern University. My research explores how we can better understand high-redshift galaxy spectra using observations and modeling. In my free time, I love to read, write, and learn about history.