Editor’s Note: Astrobites is a graduate-student-run organization that digests astrophysical literature for undergraduate students. As part of the partnership between the AAS and astrobites, we occasionally repost astrobites content here at AAS Nova. We hope you enjoy this post from astrobites; the original can be viewed at astrobites.org.
Title: SPLUS J142445.34-254247.1: An r-process-enhanced, Actinide-Boost, Extremely Metal-Poor Star Observed with GHOST
Authors: Vinicius M. Placco et al.
First Author’s Institution: NSF’s National Optical-Infrared Astronomy Research Laboratory (NOIRLab)
Status: Published in ApJ
Have you ever looked at the periodic table and wondered where all the elements come from? Nearly all of the hydrogen, helium, and some of the lithium present in the universe formed in the first three minutes after the Big Bang. Beyond that, most of the elements (those that are heavier than helium are called metals by astronomers) were created in nuclear reactions that take place in the heart of stars. Up through iron, the formation processes happened systematically, where heavier elements were formed from the fusion of lighter elements. Beyond iron, about half of the elements are created by a slow and gradual process of capturing neutrons (called the s process, with s for slow).
Elements beyond iron are created in extreme events, such as stellar explosions (see Figure 1 for an overview). Such cataclysmic events cause neutrons to rapidly bombard atomic nuclei, forming heavier elements. This rapid capture of neutrons is called the r process (with r for rapid). There is a constant exchange of elements between the stars and the gas surrounding them. This eventually leads to the formation of more stars that are enriched in metals, setting up different generations of stars.
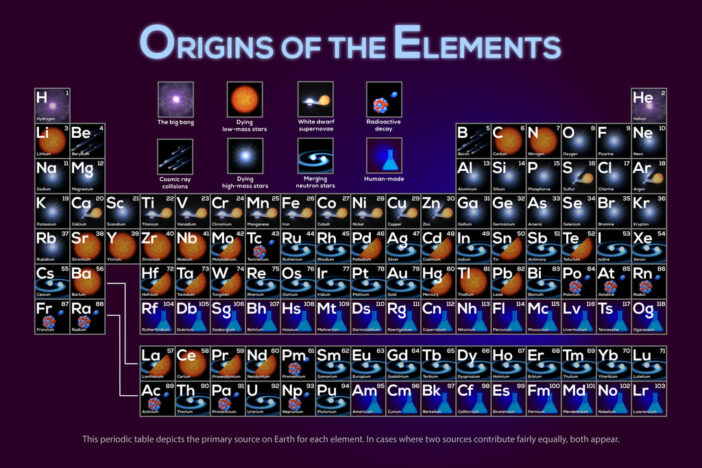
Figure 1: The astronomer’s periodic table indicates the elements’ astrophysical origin. [NASA’s Goddard Space Flight Center]
If we obtain high-resolution spectroscopy of a star and map the abundances of all its elements, it would paint a picture of the events that led to its formation. In today’s article, the authors try to understand how stars were formed in the halo of the Milky Way by recreating the formation scenario based on the fingerprints in the high-resolution spectrum obtained from a Milky Way halo star called SPLUS J142445.34–254247.1, or SPLUS J1424−2542 for short.
Recreating the Formation Scenario
The star’s chemical abundances are determined from absorption features in its spectrum. If a particular element is present, it will absorb the starlight passing through the cold gas at a characteristic wavelength, and the extent of absorption can be used to determine how much of the element is present in the star. Looking at the elemental abundances of SPLUS J1424−2542, the star showed signs of being poor in iron (atomic number Z = 26) but being enhanced in elements with atomic numbers 26 < Z < 38 compared to the standard values measured from the Sun. This indicates the star formed from a gas cloud polluted by two distinct populations of stars in a multi-enriched process.
The abundances of the heavier elements indicate that the primary process involved in the formation is the r process. This is not uncommon for old stars in the galactic halo. The s-process elements are formed from the death of low-mass stars, which would not have occurred when such old stars were formed in the halo. Most elements in halo stars are created through the r process, even those typically formed by the s process. However, the authors found that certain elements, such as strontium, do not agree with the predicted values from either the r process or s process (Figure 2). Other elements, such as barium, are overproduced, indicating contributions from both s and r processes. The team also found an overabundance of thorium, which could indicate a possible contribution from a separate r-process event. Thus, this star is metal poor with enhanced heavy elements produced by r-process events.
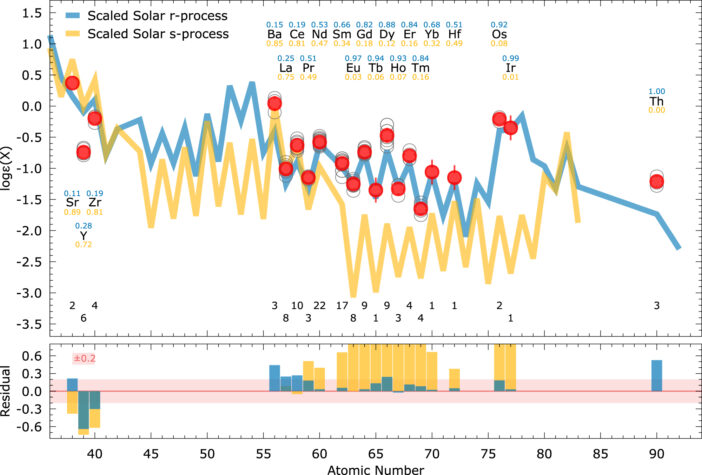
Figure 2: Observed abundances (red circles) with the expected values from the r process (blue line) and s process (yellow line). Most points lie on the blue line, indicating a more significant contribution from the r process. [Placco et al. 2023]
Mysterious Circumstances Prevail
The authors derived the star’s kinematic properties (such as orbital dynamics, velocities, etc.), which are displayed in Figure 3. They found that the proposed formation scenario and the derived kinematics do not connect the star with any known structure in the Milky Way. This highlights that a distinct star formation mechanism may occur in the galactic halo. We must continue studying similar stars with high-resolution spectroscopy to help us understand the formation of old stars in the Milky Way halo.
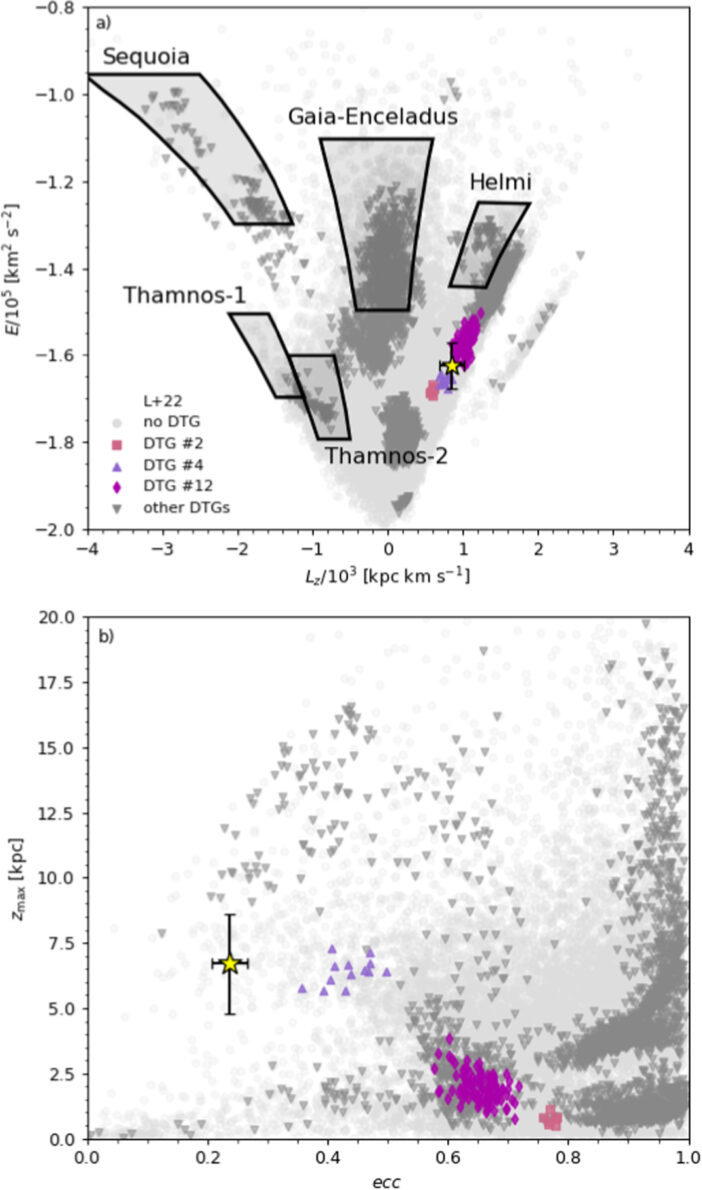
Figure 3: The yellow star indicates the star studied in this article. The upper panel shows it does not fall into the expected Milky Way streams. The bottom panel shows that it has distinct properties from other stars in a similar position on the upper panel. [Placco et al. 2023]
About the author, Archana Aravindan:
I am a PhD candidate at the University of California, Riverside, where I study black hole activity in small galaxies. When I am not looking through some incredible telescopes, you can usually find me reading, thinking about policy, or learning a cool language!