Editor’s Note: Astrobites is a graduate-student-run organization that digests astrophysical literature for undergraduate students. As part of the partnership between the AAS and astrobites, we occasionally repost astrobites content here at AAS Nova. We hope you enjoy this post from astrobites; the original can be viewed at astrobites.org.
Title: Formation History of HD106906 and the Vertical Warping of Debris Disks by an External Inclined Companion
Authors: Nathaniel Moore et al.
First Author’s Institution: Georgia Institute of Technology
Status: Published in ApJ
When early astronomers theorized how planets formed, they often used the solar system as a model, mainly because that was all we had observationally available at the time. The thing is, the solar system is pretty “well behaved” — the planets are more or less in the same orbital plane, and their orbits are not too eccentric (i.e., they are closer to being circles than ellipses). However, as more exoplanets are found, astronomers begin to question their ideas for how planets formed. The binary system HD 106906, for example, has an asymmetrical debris disk and a planet that is not in the same plane as the disk and is separated from the stars by 730 astronomical units (au; for reference, Earth is 1 au away from the Sun). This system has an unusual architecture, and the authors of today’s article try to theorize how this system formed. Understanding the formation of an usual system like this one allows us to expand our knowledge of planet formation beyond the simplicity of such well-behaved systems such as our own!
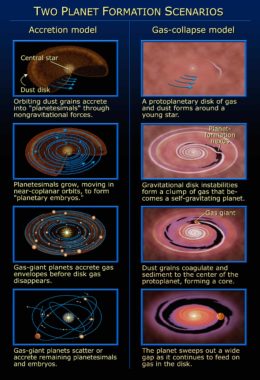
Figure 1: The two possible scenarios for planet formation: accretion model (“bottom-up”) and gravitational instability (“top-down”). Click to enlarge. [NASA and A. Feild (STScI)]
The authors explore this idea using N-body simulations (a simulation of how bodies interact over a period of time) of the system combined with simulations of how the observational data would look for this scenario. They then compare the simulations to real observations.
Companion and Disk Interactions
The authors first try to determine whether the HD 106906 system has been like this for a long time or if its current configuration is the result of a recent event. To do this, they simulate different variations of the planet’s eccentricity, inclination, and semi-major axis. For the simulations, they include the effects of radiation pressure. They also use two different central body configurations: one with a binary star system and another with a single central body and an extra J2 potential term, which emulates the binary system but is more computationally efficient. The main results from these simulations are shown in Figure 2.
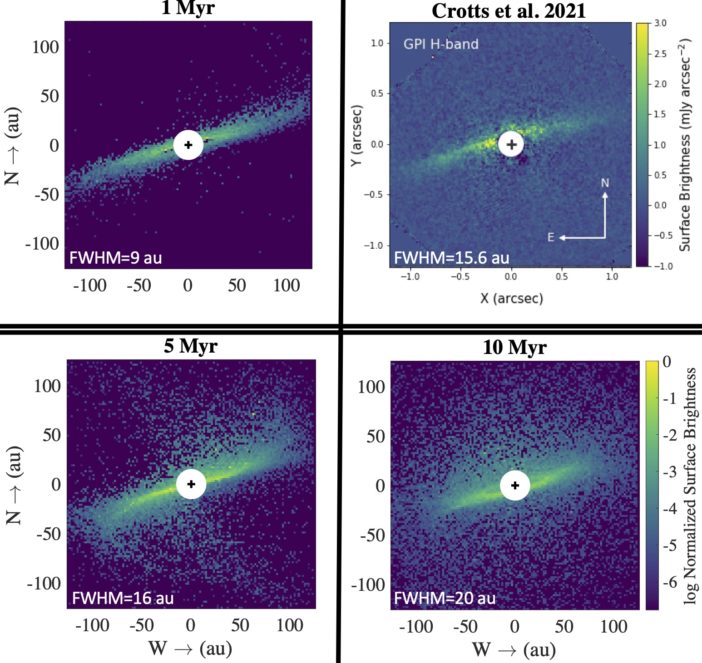
Figure 2: The simulations at 1 million years (top left), 5 million years, (bottom left) and 10 million years (bottom right) compared to the Crotts et al. 2021 original observations. After a million years, the simulated disk is very similar to the real image. By 5 and 10 million years, the appearance (size and brightness) of the disk exceeds the observational constraints. [Moore et al. 2023]
Knock, Knock. Who’s There?
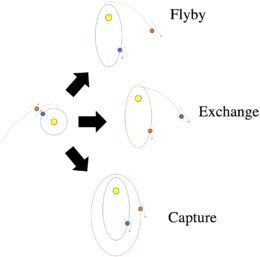
Figure 3: The free-floating planet, represented in red, can simply “fly by” the system, leaving the original configuration mostly unchanged; be exchanged with the original planet (blue), which then gets ejected; or be captured into the system. [Moore et al. 2023]
The team’s final results are shown in Figure 4. From the figure, we can see that a few outcomes in which either the free-floating planet stays in the system or the native planet stays in the system agree with observations. The authors conclude that an encounter with a free-floating planet is a possible explanation for the current architecture of this system. The close encounter only reproduces observational results 0.2% of the time, but this system is quite unusual — so a low probability of a system forming like this is expected!
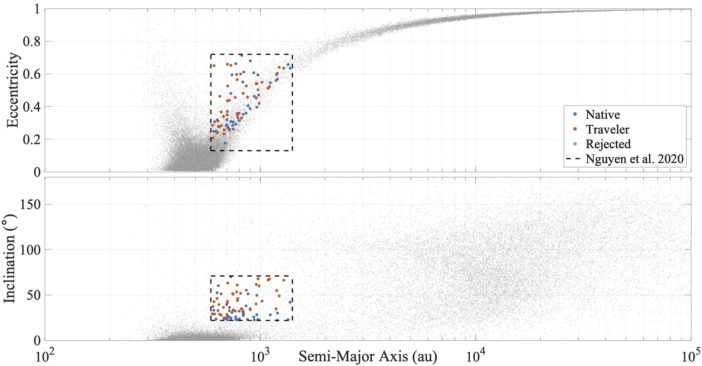
Figure 4: The final results of the close encounter simulations of the free-floating planet and the HD 106906 system. The dots within the dashed square fall within the expected observational constraints of the companion (i.e., that agree with current estimates for the orbital eccentricity, semi-major axis, and inclination of the companion). The blue dots represent the outcomes that agree with observations where the native planet remains bound to the system. The red dots represent the outcomes where the free-floating planet remains bound to the system. The gray dots represent the other parameters of the 100,000 simulations. [Moore et al. 2022]
About the author, Clarissa Do O:
I am a third-year physics graduate student at UC San Diego. I study exoplanet orbital dynamics and also work on exoplanet instrumentation. My current work is on the adaptive optics upgrade of the Gemini Planet Imager 2.0, an instrument that aims to directly image and characterize exoplanets.