Editor’s Note: Astrobites is a graduate-student-run organization that digests astrophysical literature for undergraduate students. As part of the partnership between the AAS and astrobites, we occasionally repost astrobites content here at AAS Nova. We hope you enjoy this post from astrobites; the original can be viewed at astrobites.org.
Title: Pre-Supernova Alert System for Super-Kamiokande
Authors: The Super-Kamiokande Collaboration
First Author’s Institution: Institutions affiliated with the Super-Kamiokande Collaboration
Status: Published in ApJ
Let me start with a fun fact that you might have heard before: there are literally trillions of neutrinos flying through you every second while you are reading this. You might have noticed that you don’t feel these neutrinos at all (see also this article). It gets really weird knowing that most neutrinos casually fly through Earth without noticing the thousands of kilometers of planet they are passing through, and it’s even weirder to consider that these particles were only first detected in the 1950s.
This immediately shows the problem with neutrinos: they really don’t like to talk to us. In a more scientifically correct way, we can say that neutrinos are weakly interacting. In fact, the only forces that have any sway on them are gravity and the weak force, the weakest of the four fundamental forces of nature. Of course, this doesn’t stop physicists from looking for neutrinos anyway. To do so, researchers build huge detectors, usually deep underground to shield the detectors from other cosmic radiation. These detectors are typically, although not always, filled with thousands of metric tons of heavy water that is surrounded by tens of thousands of light detectors in order to have a chance of seeing a few neutrinos.
Now, how does a large pool of heavy water (no, you can’t swim in it) and a bunch of extremely sensitive light sensors help us detect a particle that doesn’t even care about an entire planet of material? This astrobite explains it swimmingly (pun intended). In short, neutrinos can only interact on very short distances and mainly do so with neutrons, so a good detector contains a large number of neutrons. We can provide an abundance of neutrons by either using a very dense material that naturally increases the amount of neutrons per volume, or by using materials that have more neutrons per atom. Heavy water fulfills the latter requirement. The light emitted by a neutrino interacting with a neutron is then seen by the light detectors, helping us find our neutrinos. Or at least a few of them — most still just fly through the detector without doing anything.
Take It with a Grain of Gadolinium
Today’s article discusses an improvement made to the Super-Kamiokande detector and how that improvement helps astronomers predict when a supernova might occur. In 2020, researchers added some 13 tons of gadolinium sulfate octahydrate to the water of the detector. Of course, they didn’t just add tons of a random molecule — gadolinium is around 100,000 times more likely to interact with neutrinos than hydrogen. Overall, this addition helps the the detector capture twice as many neutrinos as well as harder-to-detect low-energy neutrinos.
It is exactly these lower-energy neutrinos that are relevant to predicting supernovae. In the cores of stars that are expected to go supernova, more and more violent nuclear reactions occur as the star approaches its end. In these last moments, just hours before the supernova, silicon atoms start to fuse and neutrinos are emitted as a result. With tons of new gadolinium molecules in the Super-Kamiokande detector, some of the neutrinos from this silicon fusion could be detected. For a well-known star like Betelgeuse, the silicon fusion would start about 10 hours before the star goes supernova. This would give astronomers an extra 10-hour warning, allowing them time to point every telescope and detector they can get their hands on towards the supernova candidate. The authors of today’s article predict how we would see these neutrinos coming in, shown in Figure 1.
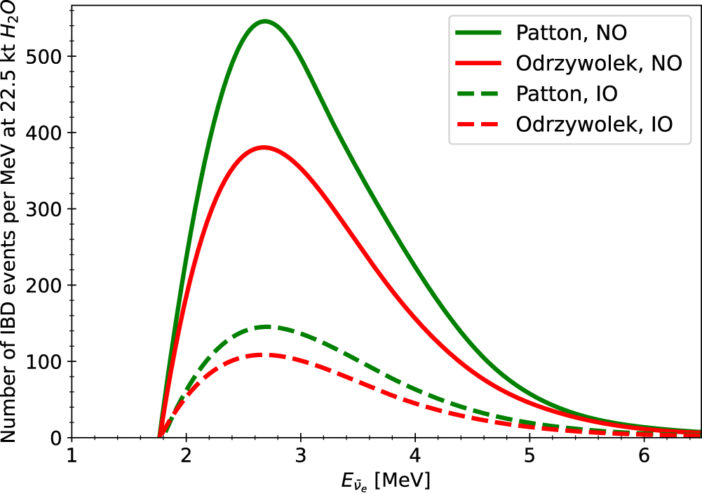
Figure 1: Predicted number of neutrino detections in the Super-Kamiokande detector (y axis) per neutrino energy (x axis) in mega electron volts (MeV). The prediction is based on calculations with a Betelgeuse-like star and the neutrinos are expected to come mainly from silicon fusion adding up in the last 10 hours of the star’s life. The different colors highlight the different simulations used, while the full and dashed lines show the neutrinos expected from normal (NO) and inverted (IO) mass ordering, respectively. [Super-Kamiokande Collaboration 2022]
- The star’s mass: Less-massive stars have a lower probability of emitting detectable neutrinos, simply because they will emit fewer neutrinos overall.
- The distance to the star: The detectable neutrinos are less likely to be observed if the star is farther away because the neutrinos are spread farther apart.
- Evolution of the star: Stars live their lives in very different ways. For example, stars with more metals will behave differently, which influences when and how many detectable neutrinos they will spew out.
- Neutrino mass ordering: Neutrino masses aren’t well constrained, since we have no reliable measurements of their masses. It is even uncertain which neutrino flavors have more or less mass, so the authors assume a normal and an inverse mass ordering based on these different neutrinos (see also Figure 1).
The detector’s sensitivity could also be increased considerably by adding more of the gadolinium molecule to the detector’s water.
All in all, the authors are fairly confident that the Super-Kamiokande detector can tell them whether a star up to nearly 2,000 light-years away from Earth will go supernova, giving us a warning several hours before the star finally and spectacularly kicks the bucket.
Original astrobite edited by Alice Curtin.
About the author, Roel Lefever:
Roel is a first-year PhD student at Heidelberg University, studying astrophysics. He works on massive stars and simulates their atmospheres/outflows. In his spare time, he likes to hike/bike in nature, play (a whole lot of) video games, play/listen to music (movie soundtracks!), and to read (currently The Wheel of Time, but any fantasy really).