Editor’s Note: Astrobites is a graduate-student-run organization that digests astrophysical literature for undergraduate students. As part of the partnership between the AAS and astrobites, we occasionally repost astrobites content here at AAS Nova. We hope you enjoy this post from astrobites; the original can be viewed at astrobites.org.
Title: Long Dark Gaps in the Lyβ Forest at z<6: Evidence of Ultra Late Reionization from XQR-30 Spectra
Authors: Yongda Zhu et al.
First Author’s Institution: University of California, Riverside
Status: Published in ApJ
The who, what, when, and where of reionization are unresolved questions that have important implications for our understanding of the cosmos. This final phase transition of the universe from neutral to ionized encompasses a variety of dramatic changes, as large-scale structures formed and evolved and the first stars and galaxies began to light up the universe. While shining a light on the history of reionization would also help uncover the history of how objects in the universe emerged and grew, this epoch is fundamentally dark.
However, we do have the basics of the why down: before the epoch of reionization, the universe was mostly filled with neutral hydrogen gas. Then, as the first stars, galaxies, and quasars began to form and started shining, these objects emitted high-energy photons that ionized the neutral gas around them. The ionizing radiation kicked out electrons from neutral hydrogen atoms until eventually most of the gas in the universe became ionized.
One key part of gaining a complete understanding of reionization is the when — precisely when did it begin and end, and how rapidly? There are a few main methods for probing these transition points, all of which suggest the process occurred early on, with a midpoint at roughly redshift z ~ 8 (600 million years after the Big Bang) and an endpoint somewhere around z ~ 5.5–6 (1 billion years after the Big Bang).
Absorbing It All
Many of the techniques used to trace reionization, including those used in today’s article, involve the Lyman series transitions of hydrogen, especially the Lyman-α (n=2 to n=1) transition. One such technique relies on observations of quasars (active supermassive black holes in the centers of galaxies and among the brightest objects in the universe) during the epoch of reionization. By observing distant quasars, we can understand the gas content in the universe along that line of sight using the presence and absence of Lyman-α emission and absorption compared to typical quasar spectra, which are fairly well understood and have strong signals.
As emission from a quasar travels through material between the quasar and the observer, some emission gets intercepted by gas clouds along the way, which can absorb the emission and produce a Lyman-α absorption line at a wavelength determined by the redshift of the gas cloud. However, at redshifts before the end of the epoch of reionization, the primarily neutral gas in the way will be very opaque at this wavelength, as photons with wavelengths (energies) near Lyman-α struggle to pass through the neutral hydrogen, which is optically thick enough to suppress observed emission nearly completely. This optical thickness to high-energy photons results in a contiguous region of strong absorption in the spectrum that is known as a dark gap (see Figure 1).
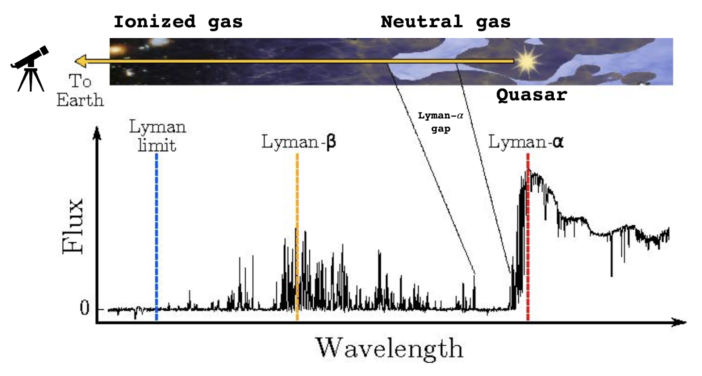
Figure 1: Example spectrum of a distant quasar with ionizing radiation emitted from the quasar intercepted by neutral gas along the line of sight. Emission lines directly from the quasar are marked by dashed colored lines. An over-dense patch of neutral gas causes a gap in the Lyman-α emission as nearly all of the flux from the quasar is absorbed. [Adapted from Figure 7 in An Introductory Review on Cosmic Reionization by John H. Wise]
Filling In the Gaps
These dark gaps could be caused by several different processes within reionization. For one, the ionizing background radiation itself could have some fluctuations, though the authors explain that their results and other recent results disfavor this scenario as it doesn’t imply sufficient neutral gas at later times. Alternatively, the prevalence of so-called “islands” of neutral gas, like pockets of Lyman photon absorption, could be the cause. Lastly, reionization could simply end later in the history of the universe, meaning more neutral gas is available to absorb high-energy photons at lower redshifts.
These scenarios are difficult to disentangle with Lyman-α gaps alone. One solution, as presented in today’s article, is to use a Lyman transition with a slightly shorter wavelength that passes through neutral gas slightly more easily (i.e., the neutral gas has a lower optical depth at that wavelength). The authors use this technique of tracing long dark gaps in quasar spectra but apply it to another Lyman transition, Lyman-β (n=3 to n=1). In order to study the dark Lyman-β gaps, the authors analyze spectra of a sample of epoch of reionization quasars at z > 5.5. Within each spectrum, they map out the dark gaps of Lyman-β absorption, the length of the gaps, and the redshift evolution of the gaps. As shown in Figure 2, one quasar spectrum in particular had a uniquely long dark gap down to z ~ 5.5. Within that line of sight, the authors found a low-density region of galaxies, which supports the idea that highly opaque sight lines are associated with galaxy underdensities. This makes sense: in areas with fewer galaxies to ionize their surroundings, there is more remaining neutral gas.
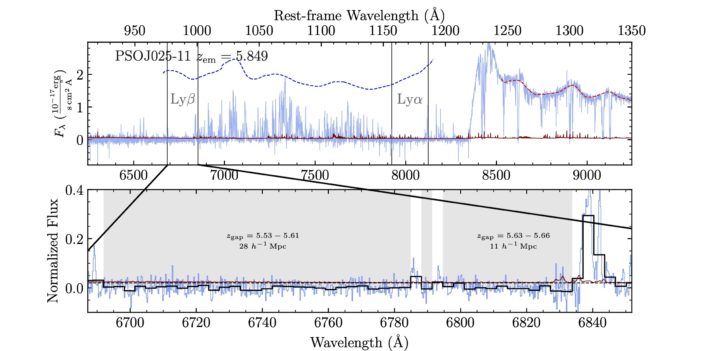
Figure 2: The light blue line in the top panel shows the spectrum for the quasar, and the dashed curve shows the predicted emission from the quasar in the absence of absorption from gas in front of the quasar. The region over which Lyman-β gaps were searched for is labeled, as is the corresponding Lyman-α forest for the same redshift range. The bottom panel shows a zoom-in of the Lyman-β gap region, with gaps (flux lower than the dashed threshold) shaded in gray. The the dark gap between z = 5.53 and 5.61 is the longest gap detected in the survey. [Adapted from Zhu et al. 2022]
Given the distinction enabled by Lyman-β data, the authors propose the best-fit scenario for their dark gap sample is late reionization, with the epoch of reionization ending at z ~ 5.3. They demonstrate that rapid late-reionization models, specifically those with a fraction of neutral gas > 5% at z = 5.6, are consistent with the observations. Looking ahead, these dark Lyman-β gaps and future large samples of quasar spectra with gaps can help fill in the gaps in our knowledge of the timing of reionization.
Original astrobite edited by Jana Steuer.
About the author, Olivia Cooper:
I’m a second-year grad student at UT Austin studying the obscured early universe, specifically the formation and evolution of dusty star-forming galaxies. In undergrad at Smith College, I studied astrophysics and climate change communication. Besides doing science with pretty pictures of distant galaxies, I also like driving to the middle of nowhere to take pretty pictures of our own galaxy!