Editor’s note: Astrobites is a graduate-student-run organization that digests astrophysical literature for undergraduate students. As part of the partnership between the AAS and astrobites, we occasionally repost astrobites content here at AAS Nova. We hope you enjoy this post from astrobites; the original can be viewed at astrobites.org.
Title: Indication of a Pulsar Wind Nebula in the hard X-ray emission from SN 1987A
Authors: Emanuele Greco et al.
First Author’s Institution: University of Palermo, Italy
Status: Accepted to ApJL
In 1987 astronomers witnessed the closest supernova in almost 400 years, subsequently called SN 1987A. At only 51.4 kiloparsecs (or about 167,000 light-years), SN 1987A’s home is in the Large Magellanic Cloud, and it was visible in the Southern Hemisphere with the naked eye for a few months before it faded. But one question that remains unanswered is what kind of object was left behind. The original star that created SN 1987A was a blue supergiant, which would have left behind either a black hole or a neutron star. Yet even with decades of observations by many telescopes spanning the electromagnetic spectrum, its nature has yet to be confirmed.
Why are astronomers still trying to figure out what was left behind in SN 1987A? One reason is that it would let us learn more about neutron star and black hole formation and the mechanics of supernovae. Another reason is that if this leftover object happens to be a pulsar, a neutron star that emits radio (and potentially X-ray or gamma-ray) pulses, then we would be able to observe its very early, formational years, which we know very little about. Recent work (like that discussed in this astrobite) suggests that a neutron star is the likely remnant, but we can’t say for sure. The authors of today’s paper attempt to confirm once and for all that the leftover remnant of SN 1987A is a neutron star.
Look with Your X-ray Eyes
To determine the nature of the object at the center of SN 1987A, the authors use X-ray observations taken between 2012 and 2014 by the Chandra X-ray Observatory, which observes X-ray photons between 0.1 and 10 keV, and NuSTAR X-ray telescope, which observes X-ray photons between 3 and 79 keV (though the full range of each telescope is not necessarily used in the analysis). The images of SN 1987A from these telescopes are shown in Figure 1, with redder colors representing more photons detected.
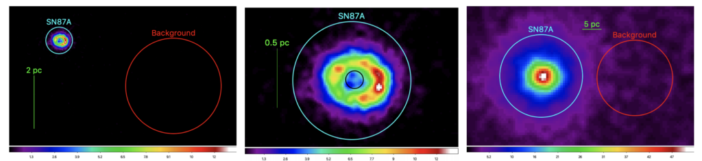
Figure 1: X-ray images of SN 1987A where redder colors represent more X-rays. Left: Image from the Chandra X-ray Observatory from 0.1–8 keV. The cyan circle shows SN 1987A, and the red circle shows the noise level of the background X-rays. Since the background is almost completely black, there is very little noise. Center: A zoom-in of the left panel. The X-ray dim center of SN 1987A is shown by the black circle in the center. Right: The NuSTAR image from 3–30 keV. SN 1987A is circled again in cyan, and the slightly noisier background is circled in red. SN 1987A still clearly stands out above the background. [Greco et al. 2021]
The second model is the same as the first, but it also includes a model for a highly absorbed pulsar wind nebula (PWN). PWNs are astronomical winds of charged particles accelerating close to the speed of light around a pulsar, and they are known to give off high energy X-rays. Being highly absorbed means that very few of the X-rays emitted by a PWN would escape the gas and dust that make up the supernova remnant of SN 1987A; most are reabsorbed instead. The authors compute the residuals by subtracting these best-fit models from the X-ray spectra, shown in the bottom panels of Figure 2. The closer these residuals are to zero, the better the model. If this second model fits much better than the first, then the authors can say that there is very likely a PWN, and hence a neutron star, at the center of SN 1987A.
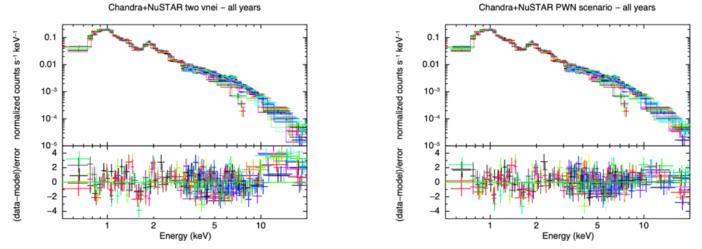
Figure 2: Combined X-ray spectra showing the number of X-ray photons observed in each energy bin of all Chandra and NuSTAR observations over the span of three years with different colors for each observation. Spectra from Chandra span 0.5–8 keV, and spectra from NuSTAR span 3–20 keV. The bottom panels show the residuals, or the spectra after the best-fit models have been subtracted off. Left: Spectra with a best-fit model containing just two thermal components. One can see that there is an excess of photons at energies higher than 10 keV in the bottom panel, as shown by the points all above the bright green zero line. Right: Same as the left, but the best-fit model has an absorbed pulsar wind nebula component in addition to the two thermal components. The excess X-rays at energies > 10 keV appear to be accounted for here. [Greco et al. 2021]
So What’s at the Center?
Unfortunately, the authors were unable to conclusively answer that question. They found that the model that includes a PWN is statistically slightly better than the one without (shown by the better residuals in Figure 2 at energies > 10 keV), but not so much that they can say anything definitively. They were able to come up with a way that the higher energy X-rays might be produced without a PWN, but it involves an extremely energetic shockwave expanding steadily outwards at the fastest speeds allowed with no slowing down. While this is possible, it is an unlikely physical scenario compared to just having a neutron star at the center of SN 1987A.
Despite the uncertainty still surrounding the central object of SN 1987A, all is not lost! The authors also did some simulations showing that, if there really is a PWN at the center of SN 1987A, then by the 2030s, fewer of the lower energy X-ray photons will be absorbed, allowing these photons to be more easily detectable with Chandra or potential future X-ray observatories. So while the nature of what SN 1987A left behind remains a mystery for now, we are getting increasingly closer to solving it.
Original astrobite edited by Anthony Maue.
About the author, Brent Shapiro-Albert:
I’m a fourth year graduate student at West Virginia University studying various aspects of pulsars. I’m a member of the NANOGrav collaboration which uses pulsar timing arrays to detect gravitational waves. In particular I study how the interstellar medium affects the pulsar emission. Other than research I enjoy reading, hiking, and video games.