Editor’s note: Astrobites is a graduate-student-run organization that digests astrophysical literature for undergraduate students. As part of the partnership between the AAS and astrobites, we occasionally repost astrobites content here at AAS Nova. We hope you enjoy this post from astrobites; the original can be viewed at astrobites.org.
Title: Asteroseismic constraints on the cosmic-time variation of the gravitational constant from an ancient main-sequence star
Authors: Earl Patrick Bellinger, Jørgen Christensen-Dalsgaard
First Author’s Institution: Aarhus University, Denmark
Status: Accepted to ApJL
A Cosmic Constant
The gravitational constant, G, is one of the core fundamental constants of physics, appearing in Newton’s laws of gravitational motion, and therefore in the fundamental theory of gravity. While people historically questioned whether it truly is a constant, Einstein’s theory of general relativity states that G must be constant no matter where in space, or time, you find yourself. However modern work in string theory, which aims to reconcile the theory of gravity with the other fundamental forces of nature, says that the gravitational “constant” can, in fact, vary over extremely long cosmic timescales.
If the gravitational constant was changing in time, we might be able to detect it in systems whose evolution has strongly relied on gravity, such as stars. If gravity was weaker in the past, that would have affected the evolution of a star, changing how it appears today. Measurements of the rate of change of G have been performed in this way using helioseismology, white dwarfs, and globular clusters, as well as studies of the cosmic microwave background. All these experiments draw the same conclusions; that G changes at a completely negligible rate (specifically, by no more than a fraction of a trillionth a year, where the universe is only 13 billion years old).
A Stellar Archaeological Dig
Today’s authors present a new test to more closely approximate the variation of G on truly cosmic timescales. The target of the authors’ study is KIC 7970740, a low-mass, solar-like star on the main sequence that is, most importantly, roughly 11 billion years old! Thanks to high-quality measurements by the Kepler space telescope, this star also has a well measured set of clear stellar pulsations that allow for an asteroseismic analysis, and it is one of the oldest stars for which this is possible.
Because this star is so low-mass, it has had a very relaxed 11-billion-year life on the main sequence, making stellar models of the star relatively simple. This makes it a perfect candidate to study historical changes in the gravitational constant G; if G changed substantially in the the universe’s history, it will have subtly affected the evolution of this star and, as a consequence, the manner in which it pulsates today (see Figures 1 & 2). For example, if G was lower in the past, gravity will have been weaker. As a result, hydrostatic equilibrium will cause the stellar radius to be larger, which increases the star’s energy output (or luminosity). More luminous stars burn faster, changing the composition of the stellar core, which in turn affects how pulsation frequencies appear on the surface.
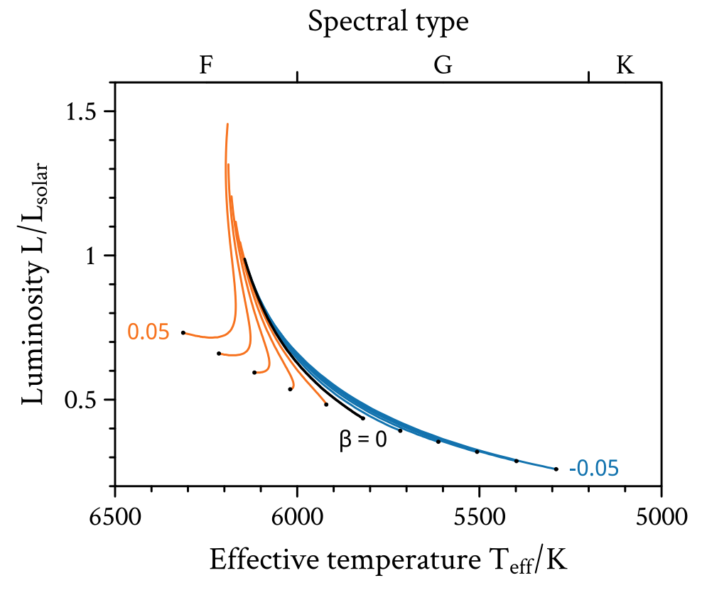
Figure 1: Showing the theoretical evolution on a HR-diagram of a low-mass star for varying degrees of change in the gravitational constant G. The black dots indicate the beginning of the star’s life on the main sequence, after which the star evolves up and to the left. The value β indicates the overall fractional change in G in the models. [Bellinger & Christensen-Dalsgaard 2019]
Modelling the Stellar History
To study the history of G, then, the authors study the history of KIC 7970740, by tweaking the star’s parameters in a stellar model and comparing the result to the observed pulsation frequencies, which are also modelled. Included in these models is a parameter β, representing the fractional change in the gravitational constant, and also the age of the universe, t0, which are both allowed to vary.
By fitting their evolutionary model to the asteroseismic data (in a process that took 6 months to run!), the authors find a fractional rate of change of G of (2.1 ± 2.9) × 10-12 yr-1, or two trillionths a year.
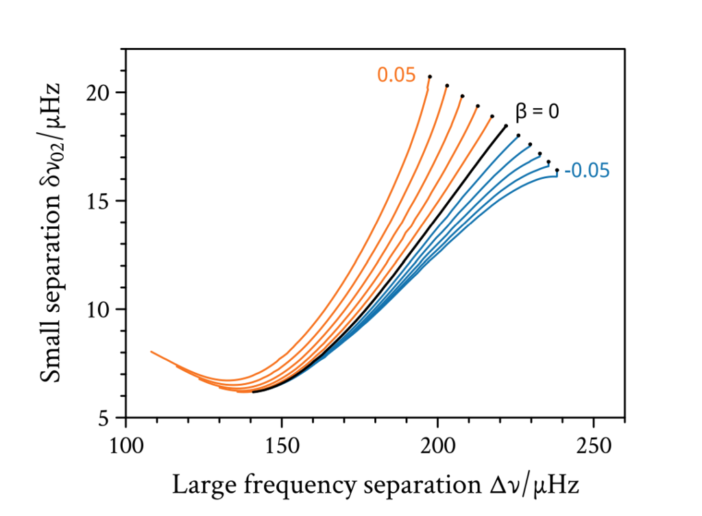
Figure 2: The same as Figure 1, now showing the evolution of the star in terms of two fundamental asteroseismic observables, Δν (the separation between oscillations of different overtones) and δν02 (the separation between oscillations of radial degree 0 and 2). [Bellinger & Christensen-Dalsgaard 2019]
Conclusions
While this result is uncertain, it is in line with previous studies on the change in G from stars — but measured over an extended age range that almost spans the full history of the universe. It is worth noting that the stellar parameters recovered for the star agree with independent studies, indicating that their model fits well and giving additional credibility to these results.
With this result, investigation of G is far from over. With the development of this technique it will become possible to apply it to an ensemble of stars, hopefully yielding a stronger result and/or highlighting any model dependencies that may have affected this result. Continuing this type of research will, hopefully, continue to improve synergies between stellar astrophysics and the most fundamental studies of the universe.
About the author, Oliver Hall:
Oliver is a final year PhD student at the University of Birmingham, UK. He’s a part of their Sun, Stars & Exoplanets research group with a focus on asteroseismology, the study of stellar pulsations, and what it can tell us about stellar populations. When not doing research he enjoys playing piano, hiking, and not moving from the sofa all weekend with a good book, show, or game.