Big news: the Laser Interferometer Gravitational-Wave Observatory (LIGO) has detected its first gravitational-wave signal! Not only is the detection of this signal a major technical accomplishment and an exciting confirmation of general relativity, but it also has huge implications for black-hole astrophysics.
What did LIGO see?
LIGO is designed to detect the ripples in space-time created by two massive objects orbiting each other. These waves can reach observable amplitudes when a binary system consisting of two especially massive objects — i.e., black holes or neutron stars — reach the end of their inspiral and merge.
LIGO has been unsuccessfully searching for gravitational waves since its initial operations in 2002, but a recent upgrade in its design has significantly increased its sensitivity and observational range. The first official observing run of Advanced LIGO began 18 September 2015, but the instruments were up and running in “engineering mode” several weeks before that. And it was in this time frame — before official observing even began! — that LIGO spotted its first gravitational wave signal: GW150914.
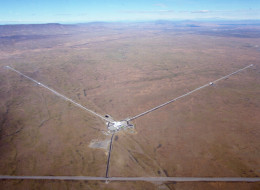
One of LIGO’s two detection sites, located near Hanford in eastern Washington. [LIGO]
Why is this detection a big deal?
This is the first direct detection of gravitational waves, providing spectacular further confirmation of Einstein’s general theory of relativity. But the implications of GW150914 go far beyond this confirmation. This detection is a huge deal for astrophysics because it’s the first direct evidence we’ve had that:
- “Heavy” stellar-mass black holes exist.
We’ve reliably measured black holes of masses up to 10–20 solar masses in X-ray binaries (binary systems in which a single neutron star or black hole accretes matter from a donor star). But this is the first proof we’ve found that stellar-mass black holes of >25 solar masses can form in nature. - Binaries consisting of two black holes can form in nature.
As we’ll discuss shortly, there are two theorized mechanisms for the formation of these black-hole binaries. Until now, however, there was no guarantee that either of those mechanisms worked! - These black-hole binaries can inspiral and merge within the age of the universe.
The formation of a black-hole binary is no guarantee that it will merge on a reasonable timescale: if the binary forms with enough separation, it could take longer than the age of the universe to merge. This detection proves that black-hole binaries can form with small enough separation to merge on observable timescales.
What can we learn from GW150914?
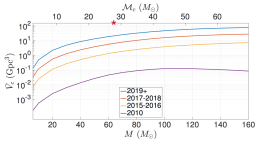
Expected increase in sensitivity for LIGO/Virgo detectors is shown as a function of total system mass (x-axis) and surveyed volume (y-axis). The red star indicates the mass of GW150914. [Abbott et al. 2016]
Another interesting conclusion about this binary system is that it probably formed in a low-metallicity environment (~ <1/2 solar metallicity). We infer this based on our current understanding of massive-star winds (which drive mass loss) and their dependence on metallicity: had the environment been high-metallicity, it is unlikely that such large black holes would have been able to form.
What can we learn from future gravitational-wave detections?
One of the key questions we’d like to answer is: how do binary black holes form? Two primary mechanisms have been proposed:
- A binary star system contains two stars that are each massive enough to individually collapse into a black hole. If the binary isn’t disrupted during the two collapse events, this forms an isolated black-hole binary.
- Single black holes form in dense cluster environments and then — because they are the most massive objects — sink to the center of the cluster. There they form pairs through dynamical interactions.
Now that we’re able to observe black-hole binaries through gravitational-wave detections, one way we could distinguish between the two formation mechanisms is from spin measurements. If we discover a clear preference for the misalignment of the two black holes’ spins, this would favor formation in clusters, where there’s no reason for the original spins to be aligned.
The current, single detection is not enough to provide constraints, but if we can compile a large enough sample of events, we can start to present a statistical case favoring one channel over the other.
What does GW150914 mean for the future of gravitational-wave detection?
The fact that Advanced LIGO detected an event even before the start of its first official observing run is certainly promising! The LIGO team estimates that the volume the detectors can probe will still increase by at least a factor of ~10 as the observing runs become more sensitive and of longer duration.
In addition, LIGO is not alone in the gravitational-wave game. LIGO’s counterpart in Europe, Virgo, is also undergoing design upgrades to increase its sensitivity. Within this year, Virgo should be able to take data simultaneously with LIGO, allowing for better localization of sources. And the launch of (e)LISA, ESA’s planned space-based interferometer, will grant us access to a new frequency range, opening a further window to the gravitational-wave sky.The detection of GW150914 marks the dawn of a new field: observational gravitational-wave astronomy. This detection alone confirms much that was purely theory before now — and given that instrument upgrades are still underway, the future of gravitational-wave detection looks incredibly promising.
Bonus
This awesome video (produced by SXS lensing) shows an actual simulation of the black-hole merger GW150914. Time is slowed by a factor of 100, compared to the actual merger. The two black holes — of 29 and 36 solar masses — warp the space-time around them, causing the distorted view.
Citation
B.P. Abbott et al. 2016 ApJL 818 L22. doi:10.3847/2041-8205/818/2/L22
3 Comments
Pingback: Live-Blog zur Gravitationswellen-Enthüllung | Skyweek Zwei Punkt Null
Pingback: Гравитационные волны: что это такое, в чем ценность открытия и как устроен детектор волн в ЛИГО | Блог издательства «Манн, Иванов и Фербер»
Pingback: Gravitational waves discovered! | daveandtelescope